Regions of Potential Existence of Free Water (Ice) in the Near-Surface Martian Ground: Results from the Mars Odyssey High-Energy Neutron Detector (HEND)
R. O. Kuzmin*, E. V. Zabalueva*, I. G. Mitrofanov**, M. L. Litvak**, W. V. Boynton***, and R. S. Saunders****
* Vernadsky Institute of Geochemistry and Analytical Chemistry, Russian Academy of Sciences, ul. Kosygina 19, Moscow, 117975 Russia
** Space Research Institute, Russian Academy of Sciences, ul. Profsoyuznaya 84/32, Moscow, 117810 Russia
*** Lunar and Planetary Laboratory, University of Arizona, Tucson, AZ 85721, USA
****Jet Propulsion Laboratory, Pasadena, CA 91109, USA
Abstract - We report results of the analysis of the data on global mapping of neutron fluxes from the Martian surface, which have been obtained during the first ten months of measurements carried out by the Russian highenergy neutron detector HEND mounted aboard the American Mars Odyssey orbiter. This analysis allowed us to separate regions where free water (in ice form) prevailed in the surface layer (with a thickness of up to 2 m) of the Martian ground from regions where physically and chemically bound ground water was most likely to be the dominant form of water. The global mapping of regions with increased ice content in the ground-surface layer revealed a direct correlation with regions of polygonal terrains morphologically similar to terrestrial polygonal forms of permafrost origin. The potential content of bound water forms in the ground of circumpolar areas of the planet is also estimated.
DOI: 10.1023/B:SOLS.0000015150.61420.5b - http://www.springerlink.com/content/jt8n38156lt8q376/
-------------------------------------------------------------------------------------------------------------------------------------------------
DISTINCTIVE FEATURES OF THE STRUCTURE OF THE SURFACE LAYER
OF THE MARTIAN CRYOLITOSPHERE
The first results of the global mapping of neutron fluxes from the surface of Mars (neutron albedo) obtained by the high energy neutron detector HEND (Mitrofanov et al., 2002; Feldman et al., 2002; Boynton et al., 2002) onboard the Mars Odyssey have shown unequivocally that there is a considerable deficit of fluxes of epithermal and fast neutrons at high Martian latitudes (>50°). The subsequent analysis of the HEND measurement data, obtained during a half-year period, showed significant spatial and seasonal variations in the neutron flux at high latitudes (Litvak et al., 2002). Neutron fluxes (neutron albedo) from the surface of Mars result from the interaction of cosmic rays with chemical elements of surface material (Surkov et al., 1993; Feldman et al., 1993). High-energy neutrons are generated in the layer the thickness of which is smaller than the thickness of the layer where low-energy neutrons are produced. Epithermal neutron fluxes (with energies from 0.4 to 100 keV) measured by HEND are produced within a surface layer 1 to 2 m thick, whereas fast neutrons (with energies from 3.4 to 7.3 MeV) are emitted from a layer 0.2 to 0.3 m thick. Because the neutron mass is equal to the mass of a hydrogen atom nucleus, the presence of significant amounts of hydrogen in the Martian ground causes both neutron retardation (due to energy losses in neutron collisions) and neutron absorption by hydrogen atom nuclei, which reduces the neutron flux (Surkov et al., 1998; Feldman et al., 1993). For this reason, measurements of neutron fluxes from the surface of Mars provide a direct test for hydrogen content in the planet's ground, which is equivalent to the amount of water present in the ground in any phase and form.
The neutron flux deficit observed at high latitudes is a reliable indicator of the enrichment of the surface layer of the Martian regolith with hydrogen (Mitrofanov et al., 2002; Feldman et al., 2002), which may be mainly presented by phases and forms of water in various quantitative proportions. The fact that an increased hydrogen content in the Martian ground is observed at high latitudes reliably confirms the previous theoretical predictions of the possible stable existence of water ice in the surface regolith layer at these latitudes (Leighton and Murray, 1966; Fanale, 1976; Farmer and Doms, 1979; Kuzmin, 1983; Mellon and Jakosky, 1995). The objective of the present study is (i) to compare the global distribution of hydrogen content in the surface regolith of Mars, as derived from the HEND neutron flux measurements, with previous theoretical and observational concepts about the structure and properties of the upper horizons of the Martian permafrost (cryolithosphere) and (ii) to study spatial and temporal variations of free water (ice) content in the surface layer of the Martian ground, which were revealed during the mapping of planetary neutron albedo. We also present the results of analysis of correlations between the observed spatial distribution of the neutron flux deficit and the frequency of occurrence of some permafrost forms observed in high-resolution (1.39–7.0 m/pixel) images acquired by the Mars Global Surveyor Mars Orbiter Camera (MOC). We used for this study the results of global mapping of the Martian neutron albedo obtained between 17 February and 10 December 2002 (329° to 125° solar longitude Ls), i.e., during the winter–early summer period in the northern hemisphere of the planet.
DISTINCTIVE FEATURES OF THE STRUCTURE OF THE SURFACE LAYER OF THE MARTIAN CRYOLITOSPHERE
Because the mean global temperature of the Martian surface is close to –60°C, the upper horizons of the planet's crust are frozen through to significant depths everywhere, forming a global permafrost shell—a cryolithosphere. According to the existing model estimates (Fanale, 1976; Kuzmin, 1977, 1983; Clifford and Hillel, 1983), the cryolithosphere may be as thick as 1 to 2 km at the equator and 5 to 6 km in polar regions. This implies that water ice in the Martian permafrost is most likely to be a dominant component of volatiles and that the contents of physically and chemically bound water, CO2 ice, and gas hydrates are considerably smaller. The climatic zonality and the regional variety of physical properties of geologic materials on Mars are the major factors that have probably determined the distinctive features of the spatial distribution of ice in the planet's frozen shell.
At the present climatic conditions on Mars, the ground ice in the near-surface regolith layer is thermodynamically stable in both hemispheres only at latitudes higher than 45° (Farmer and Doms, 1979), where annual mean surface temperatures are close to or lower than the condensation temperature corresponding to the annual mean water vapor content in the atmosphere. Within the ±45° latitudinal belt, surface temperatures are considerably higher than the temperature of condensation of atmospheric moisture (196–200 K). As a result, the process of ground-ice sublimation is dominant here (on the annual mean time scale), which has led to the formation of the surface layer of desiccated frost rocks. Ground ice may persist here only at some depths: about a few meters at latitudes of ~40° (Farmer and Doms, 1979; Mellon and Jakosky, 1995) and 100 to 300 m in the equatorial zone of the planet (Kuzmin, 1983; Kuzmin et al., 1988). An opposite situation is traced at higher latitudes, and thus here the ground ice remains thermodynamically stable near the surface.
The latitudinal dependence of the occurrence and content of ice within the upper layer of the cryolithosphere (to depths of several hundred meters) is morphologically supported by specific features of the global distribution of fresh meteorite craters with fluidized and dry ejecta (Kuzmin et al., 1988). For a given dimensional class, i.e., at nearly the same excavation depths, the occurrence density of craters with fluidized ejecta (considered to be indicators of ice content in the excavated layer) is substantially higher at middle and high latitudes, whereas craters with dry ejecta (considered to be indicators of the absence of ice in the excavated layer) dominate in the ±40° latitude belt (Fig. 1). The previous global mapping of variations in the relative ice content in the Martian regolith (such content is expressed by the ratio of the diameter of fluidized crater ejecta to the crater diameter) has shown that the relative content of ground ice in the Martian permafrost at latitudes >40° (in the excavated layer as thick as several hundred meters) progressively increases with latitude (Kuzmin et al., 1988), being appreciably higher in the northern hemisphere than in the southern one (Fig. 2). It is likely that the indicated mesoscale regularities in the distribution of ice in the cryolithosphere could have also affected the properties of the surface layer itself, which is comparable in thickness (1 to 2 m) to the layer that emits epithermal neutrons measured by HEND. However, this layer differs from the underlying massive permafrost by high seasonal variability of the regime of water exchange between the atmosphere and the regolith, which stems from rapid temperature oscillations against the background of seasonally varying water content in the atmosphere. The seasonally varying regime of the surface layer temperature and the atmospheric moisture is the main cause of possible considerable temporal variations in the content of phases and forms of water in this surface layer, associated with such seasonal temperature-dependent processes as condensation–sublimation, hydration–dehydration, and adsorption–desorption.
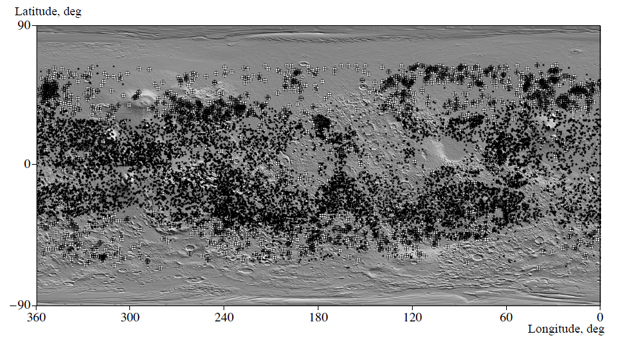
Fig. 1. The map of the observed spatial distribution of fresh meteorite craters (2 km across) with fluidized (circles) and dry (stars) ejecta over the Martian surface. Crater data are taken from (Kuzmin et al., 1988), while the map of shadow relief with a spatial resolution of 1/64 degrees based on Mars Global Surveyor Laser Altimeter data (Smith et al., 1999) is used as a background base.
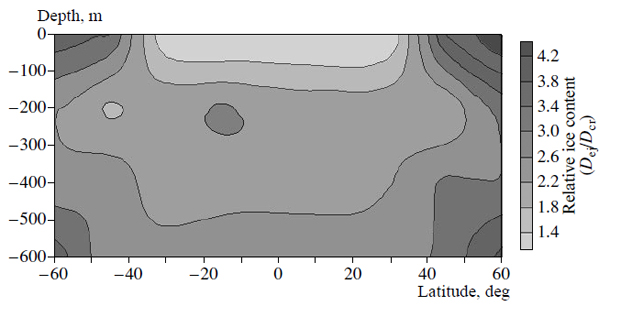
Fig. 2. The averaged meridional section of surface horizons of the Martian cryolithosphere, showing the dependence of relative ice content in the regolith on latitude and depth. The relative ice content is expressed as the ratio of the diameter of the fluidized crater ejecta to the crater diameter (Kuzmin et al., 1988).
A comparison of seasonal summer temperatures in the surface layer of the Martian ground at high latitudes with the temperature of atmospheric moisture condensation (Fig. 3) shows that the possible thickness of the seasonal sublimation layer in the northern hemisphere gradually decreases toward the pole; it is estimated to be 2 m at a latitude of 50°, 0.75 m at a latitude of 60°, and 0.25–0.30 m at a latitude of 70°. For latitudes above 70°, ice becomes stable from the immediate surface of the Martian ground. The summer temperatures were calculated for the average model of the dry ground of Mars with the following physical characteristics: albedo A = 0.25, thermal inertia I= 6.5x10-3 cal cm-2 s-1/2 K-1, and density ρ = 1.66 g/cm3. A severe cooling of the Martian surface during autumn and winter leads to the freezing of 10–15% (during northern winter) to 25% (during southern winter) of the main atmospheric component, CO2, out onto the planet's surface which results in the formation, at latitudes >56°, of the seasonal CO2 snow cover (Zurek et al., 1992). In this period of the year, when temperatures in the near-surface regolith layer at these latitudes are substantially lower than the atmospheric moisture condensation temperature, the diffusive transport of water vapor directed from the atmosphere into the regolith occurs, as well as the freezing-out of the water vapor. As a consequence, a certain amount of seasonally stable ice appears in the near-surface regolith layer. The surface layer where ice is only seasonally thermodynamically stable (the layer of seasonal permafrost) can be formed at latitudes >30° with a thickness from a few meters at middle latitudes to several tens of centimeters in the circumpolar regions. Its farthest boundary may reach a latitude of 30° in the northern and 20° in the southern hemisphere (Farmer and Doms, 1979). Mars Global Surveyor Laser Altimeter (MOLA) measurements (Smith et al., 2001), which have been carried out recently, showed that the thickness of the seasonal CO2 snow cover during Martian winter reaches 1–1.5 m at the north pole and 1 m at the south pole and the periphery of this cover may reach 56° latitude. The general structure of the uppermost surface layer (up to 3 m thick) of the Martian cryolithosphere, with its typical features just outlined, is sketched in Fig. 4.
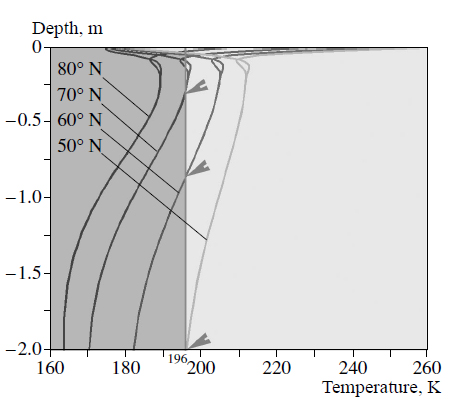
Fig. 3. The relation between seasonal summer temperatures in the surface layer of the Martian regolith (at high latitudes in the northern hemisphere of the planet) and the atmospheric water vapor condensation temperature (196 K) corresponding to the annual mean water vapor content (10mm precipitated layer). Short arrows show the base of regolith layers, within which ground ice is susceptible to sublimation in summer. Temperatures in the surface ground of Mars were estimated using the thermal model of Mars (MARSTHERM programs designed by Clifford et al., 1987).
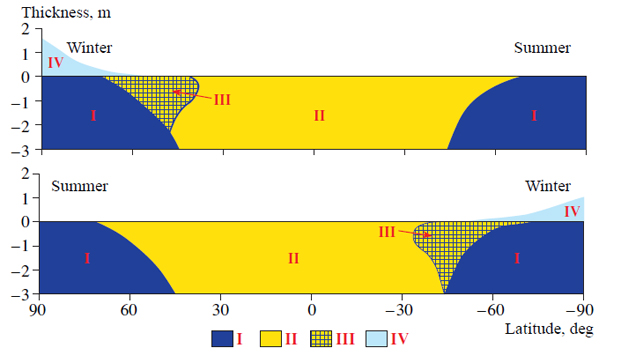
Fig. 4. The main structural features of the uppermost surface layer of the Martian cryolithosphere, typical of winter and summer periods of the year. (I) The permafrost (ice-saturated ground), in which the main volatiles are represented by water ice with secondary impurities of (1) salt solutions and gas hydrates of CO2 (seasonally) and (2) certain forms of physically and chemically bound water; (II) the permanently desiccated layer (ground frozen through without ice) containing a small amount of physically and chemically bound water and water vapor; (III) the seasonal permafrost, which arises during the autumn–winter period of the year and includes a small amount of water ice and physically and chemically bound water (Farmer and Doms, 1979); (IV) deposits in the seasonal polar cap of Mars consisting of CO2 ice with an admixture of water ice and gas hydrates of CO2 (Smith et al., 1999).
Clearly, the quantitative aspect of the temporal accumulation of ice in the uppermost surface layer depends on such factors as the latitudinal dependence of atmospheric moisture content in a given season, variations in physical properties of surface grounds, surface albedo, and terrain elevation. It is therefore quite probable that the number of phases and forms of water in the surface layer may experience seasonal variations during the Martian year. A significant deficit of epithermal and fast neutrons revealed by HEND at high Martian latitudes (Mitrofanov et al., 2002) is the first direct observational validation of theoretical predictions and results of qualitative models that pointed to the possible existence of ice-rich regolith at high latitudes of the planet.
DETERMINATION OF REGIONS OF FREE WATER OCCURRENCE (IN ICE FORM) IN THE MARTIAN REGOLITH SURFACE LAYER
At middle and low Martian latitudes, ground ice can exist only at great depths (Fanale, 1986; Kuzmin, 1983; Mellon and Jakosky, 1995), which considerably exceed the thickness of the effective layer of emission of epithermal neutron fluxes (1–2 m) measured by HEND. Therefore, at these latitudes, variations in hydrogen content, as mapped by this instrument, can probably be associated with bound water contained in hydrated minerals of the near-surface ground. However, within high latitudes, where water ice can exist in a steady state in the near-surface ground, the measured neutron fluxes are most likely to be related to the dominant content of free water in the form of ice, with a relatively lower amount of bound water (Kuzmin, 2002).
To understand the temporal variability of neutron fluxes at various latitudes of the planet, we have analyzed the HEND data obtained at various time spans during the period from winter to early summer in the northern hemisphere (Kuzmin et al., 2003). The results of our statistical analysis are presented in Figs. 5 and 6. Figure 5a shows the frequency of occurrence of various counting-rate values for epithermal neutrons (< 0.12, >0.14, and 0.12–0.14 counts per second (cps)) within a 20° latitude range for late winter in the northern hemisphere. It is remarkable that the lowest counting rates for epithermal neutrons (< 0.12 cps) are characterized predominantly by a higher occurrence frequency at latitudes higher then 60° in both hemispheres of Mars and by a very low occurrence frequency at middle and equatorial latitudes. The higher neutron counting rates (>0.14 cps) have the highest occurrence frequency mostly in mid- and low-latitude regions (Fig. 5a). In other words, these two groups of counting rate values exhibit a perfectly opposite dependence of the occurrence frequency on latitude. We consider this welldefined spatial separation of epithermal neutron counting rates as a natural separation of neutron fluxes into two independent populations, one of which (< 0.12) is presumably indicative of the presence of free water in the form of ice as a major source of hydrogen in the Martian ground, while the other (>0.14) may attest to the dominant presence of forms of bound water at low ground-ice contents. The occurrence frequency for intermediate counting rates of epithermal neutrons (0.12–0.14 cps) is more uniform at all latitudes, exhibiting, however, a small positive trend toward high latitudes of the northern hemisphere during winter time, which is probably due to the presence of seasonal CO2 snow cover. It is not improbable that these intermediate counting rates pertain to the cases when both free and bound water are present simultaneously in the Martian ground and their relative proportions can change from place to place. As follows from Figs. 5b and 5c, the mean counting rates for epithermal and fast neutrons at latitudes >60° (in both hemispheres) are also found to be considerably lower than in other regions of the planet, except during winter time, when the seasonal cover consisting of CO2 snow isolates the surface icerich regolith. As the seasonal CO2 snow cap recedes (during the spring), the zone with the lowest counting rate values for epithermal and fast neutrons progressively moves toward higher latitudes. After a complete disappearance of the seasonal CO2 snow cap in summer, a monotonic decrease of neutron counting rates is observed at latitudes >45° (see Fig. 5b). It is most likely that the trend to decrease the neutron albedo toward poles observed when there is no CO22 snow cover is due to the successive increase in the content of ground ice in the surface layer of the cryolithosphere. In this period of time, the neutron counting rates at latitudes >60° reach the values < 0.10 and < 0.12 cps for epithermal and fast neutrons, respectively.
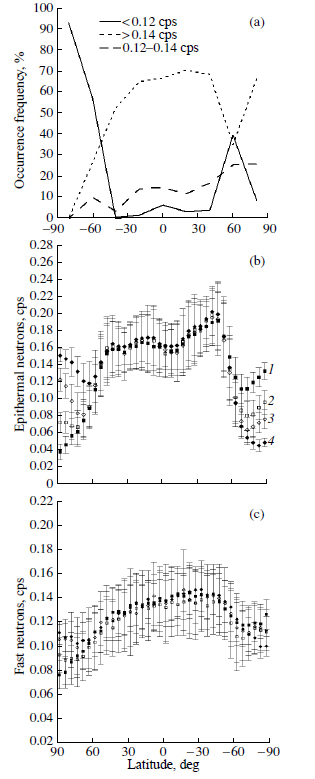
Fig. 5. (a) The occurrence frequency of various counting rates for epithermal neutrons (<0.12, > 0.14 and 0.12–0.14 cps) within a 20-degree latitude range. The dependence of average counting rates for (b) epithermal and (c) fast neutrons, respectively, emitted by the surface layer of the Martian regolith, on latitude and season in the northern hemisphere of the planet (1 is late winter; 2 is spring; 3 is the spring-tosummer period; 4 is the first half of summer). Vertical lines show a standard deviation.
In order to separate the total population of epithermal and fast neutron fluxes typical of the circumpolar regions of Mars (at latitudes >60°) from the population characteristic of the ±60° latitude belt, we made a statistical analysis of regimes of neutron fluxes mapped at different seasons (during the winter–spring period, in late spring and in summer in the northern hemisphere). The results are represented in Fig. 6 as scatter plots for counting rate values for neutrons of both energy levels. We see from Fig. 6 that epithermal and fast neutron counting rates typical of the northern and southern circumpolar regions and of the area between these regions are grouped in fairly distinct individual clusters. Figure 6 shows that in summer both circumpolar regions of Mars (at latitudes >60°) are characterized by relatively close mean values of counting rates for epithermal neutrons inside the cluster (0.062±0.022 and 0.06±0.021, respectively), whereas mean values for fast neutrons in the northern region are significantly lower (0.08±0.018) than in the southern one (0.109±0.016). The observed distinction between the fast neutron fluxes may be explained by the fact that the near-surface (20–30 cm) regolith layer in the southern circumpolar region is more hydrogen-depleted than the regolith layer in the northern region. During the winter–spring period, the range of neutron counting rates in clusters of both circumpolar regions of the planet is additionally displaced toward higher values (Fig. 6), which is due to the isolating effect of the seasonal CO2 snow-cover cap having a maximum thickness at this time of the year. During the transition period from spring to summer, when the thickness of the CO2 snow cover is minimal, the clusters of neutron counting rates become very close to each other in both circumpolar regions. In this case, the counting rates for epithermal neutrons are mostly found to be lower than 0.12 cps. Seasonal variations in the appearance of the mapped regions, where free water is supposed to be present in the Martian ground, are illustrated in Fig. 7. The observed similarity of counting rate values for epithermal neutrons in circumpolar regions (in summer) provides compelling evidence of the close proximity of ground-ice contents, on the level of average values, in the 1.5–2-m surface layer of the Martian regolith at high latitudes of both Martian hemispheres. It is remarkable that the observed behavior of neutron albedo in both circumpolar regions of the planet does not exhibit discernible correlations with various geologic units that have been earlier mapped in these regions by Viking 1 and 2 (Greeley and Gust, 1987; Scott and Tanaka, 1986). Here we also do not observe a detectable correlation with thermal inertia of the Martian ground, though the values of this parameter inferred from the results of thermal mapping of Mars (Mellon et al., 2002) are widely different in both circumpolar regions (250–500 J m-2 s-1/2 K-1 in the northern hemisphere and 60–250 J m-2 s-1/2 K-1 in the southern one). The lack of the above correlations may give evidence that the mechanisms responsible for the replenishment of the considered layer of surface ground with free water (ice) are independent of the variety of geologic units and variations in thermal inertia of the Martian ground. Most likely, these mechanisms are predetermined by climatic factors of the present or not too distant past climate of the planet. It appears that large temperature drops on the surface and inside the regolith and seasonal variations in atmospheric moisture, which are typical of these regions, are one of the principal climatic factors that determined the observed pattern of enhanced free-water content in the near-surface regolith.
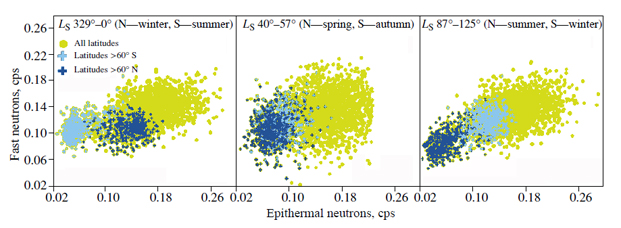
Fig. 6. Scatter plots for epithermal and fast neutrons mapped by the high- energy neutron detector (HEND) in different seasons over the entire surface of Mars (circles) and in the circumpolar regions (crosses).
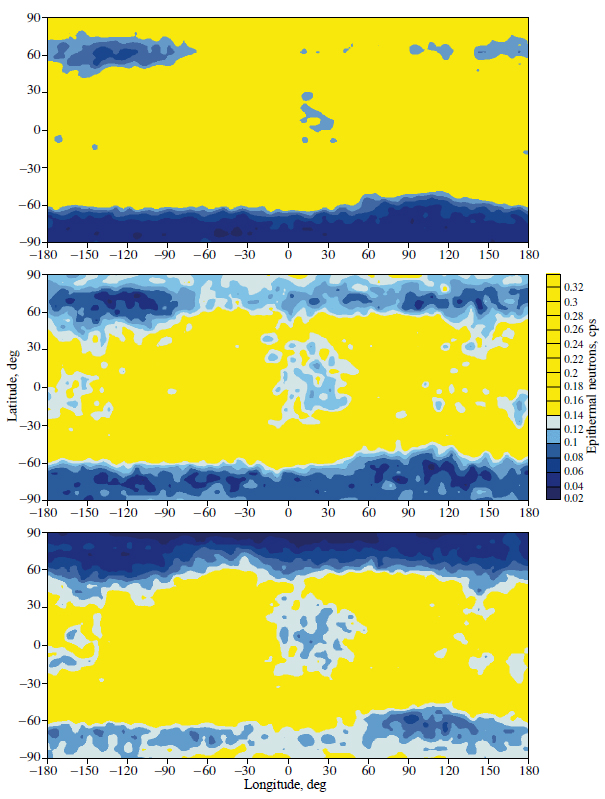
Fig. 7. Global maps of seasonal dynamics of neutron indication of the presence of free water (in the form of ice) in a 1–2 m layer of the Martian ground. The dynamics is due to the processes of condensation and sublimation of the seasonal polar cap composed of CO2 snow in the circumpolar regions. Color profiles corresponding to epithermal neutron counting rates < 0.12 cps show the occurrence regions with prevalent ground-ice content; profiles corresponding to the 0.12–0.14 cps show the regions where free and bound water are present in various quantitative proportions. The maps are constructed using the Gold Surfer program and the HEND data acquired between February 18 and December 10, 2002 (1 is the period between February 18 and April 17; 2 is the period between July 10 and September 3; 3 is the period between October 26 and December 10).
CORRELATION BETWEEN THE REGIONS WHERE FREE WATER (ICE) IS PRESENT IN THE MARTIAN REGOLITH AND THE OCCURANCE OF PERMAFROST FORMS ON MARS
To compare the revealed regions of increased presence of free water in the Martian regolith with the distribution of such permafrost landscapes as polygonal terrains we invoked the data on the global mapping of these formations (Kuzmin et al., 2003) which has been obtained using high- resolution images from the Mars Global Surveyor Mars Orbiter Camera (MOC). The global distribution of these landforms on Mars is distinctly confined to high latitudes, and they are very similar morphologically to their terrestrial analogs (Fig. 8).
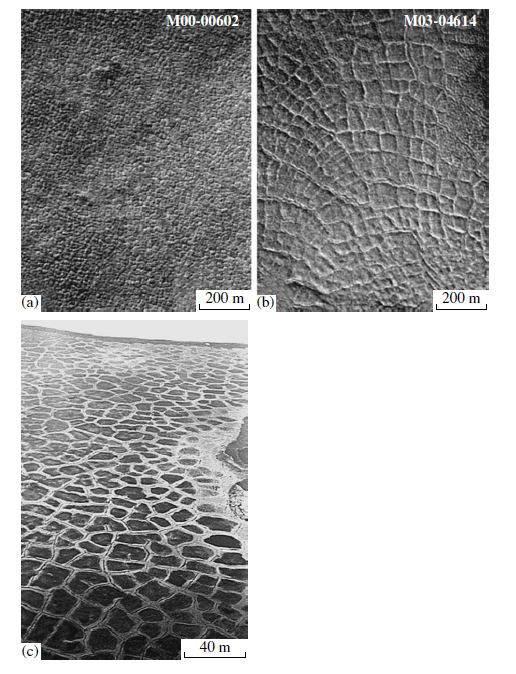
Fig. 8. Examples of polygonal terrains on Mars photographed by the MOC camera from the Mars Global Surveyor spacecraft (a, b) and terrestrial permafrost ice-veined polygonal relief in the North East Siberia, which was formed during the Pleistocene period as a result of winter frost cracking of highly ice saturated deposits: (a, b) fragments of high-resolution MOC images; (c) the surface of the alluvial plain in the midstream of the Kolyma river (Kuzmin's photo).
On the Earth, polygonal landforms are the most common form of permafrost relief in the regions of permafrost occurrence; they serve as the main morphological indicator of the presence of ice-saturated frost deposits (Romanovskii, 1977). The results of comparative analysis of Martian polygonal relief and their permafrost analogs on the Earth, which has been done recently using high-resolution MOC images (Yoshikawa, 2000; Seibert and Kargel, 2001; Kuzmin et al., 2002), point to the close morphological and morphometric similarity of these features, although the average size of polygons on Mars is, in places, 4 to 5 times greater than the size of terrestrial polygons. In view of the revealed morphological similarity between Martian and terrestrial landscapes with polygonal relief, this type of Martian terrain can be considered as a morphological indicator of the presence of ice-saturated deposits on the surface (or deposits occurring near the surface). In Fig. 9 we present a map showing the spatial distribution of regions with anomalously low (< 0.12 cps) fluxes of epithermal neutrons, corresponding to the increased ice content in a 1–2-m-thick ground-surface layer, and the regions where terrains with Martian polygonal relief are present. As can be seen from this map, a fairly distinct direct correlation exists in both hemispheres of the planet between the mapped regions of increased ground-ice content in the Martian regolith and the occurrence of terrains with polygonal relief. We see, for example, that most (90%) of the areas mapped with the use of 420 high-resolution MOC images, where polygonal landforms are present, are directly located inside the regions with enhanced ground-ice content and only 10% are outside these regions, but rather close to them. This minor divergence can be explained by assuming that the ice-rich regolith layer may be buried beneath the 1–2-m-thick surface layer, from which epithermal neutrons measured by HEND emerge.
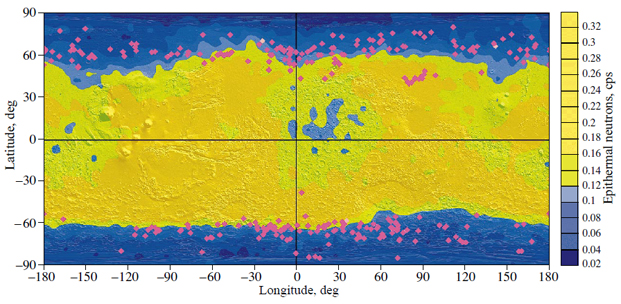
Fig. 9. The global map of Mars showing the spatial distribution of regions with enhanced free water (ice) content in the Martian ground, which are mapped by the HEND instrument in summer (for both hemispheres of the planet), and the distribution of terrains with polygonal relief (diamonds). The map of shadow Martian relief with a spatial resolution of 1/64 degrees (Smith et al., 1999) is used as a background base map. The distribution of polygonal terrains on Mars is taken from (Kuzmin and Zabalueva, 2003).
The results of statistical analysis of temporal and spatial variations of the neutron albedo of Mars imply that the regions with prevalent content of free water (in the form of ice) in the Martian ground can be rather reliably separated from the regions with dominant content of bound water forms, when the counting rates for epithermal neutrons become equal to or less than 0.12 cps. The choice of this neutron counting rate as a threshold value is supported by the existence of direct correlation between the regions of occurrence of Martian polygonal relief (as a morphological indicator of ice-bearing deposits) and the regions where free water is present in the surface ground of the planet. For this reason, the regions where neutron fluxes observed by HEND are lower than this threshold can be reliably identified as regions with the dominant content of ground ice in a 1-2-m-thick surface regolith layer. In the cases when neutron flux values turn out to be substantially higher than this threshold, we can suggest a dominant content of bound water in the Martian ground.
A similar separation between neutron indications of free and bound water in the uppermost 20–30-cm-thick near-surface layer, based on fast neutron mapping data, appears to be a more complicated problem. Although the tendency for the flux of fast neutrons to decrease which is observed at increasing latitudes in circumpolar regions (Fig. 5) may be considered indirect evidence for an increase in ice content, the relative content of ice in this layer may be significantly lower than in the underlying ground layers because of the seasonal sublimation effect. At the same time, the relative content of bound water in this layer may be much higher than at middle to low latitudes in view of the increase in mineral hydration with increasing latitude (Zolotov, 1989; Zolotov et al., 1997). As a consequence, the determination of the content of various forms and phases of water in the uppermost near-surface ground layer at latitudes >45° (to a depth of 20-30 cm) appears to be a very nontrivial problem that requires independent consideration. The existing model estimates of water content in this layer, based on gamma-ray spectrometry and highenergy neutron detector (HEND) measurements on the Mars Odyssey orbiter, give the values of about 3–5% (Boynton et al., 2002; Mitrofanov et al., 2002; Feldman et al., 2002). However, these estimates may prove to be noticeably underestimated, if we take into account the potential possibility that highly hydrated minerals (such as sulfates and chlorides) in the surface regolith of the circumpolar regions of Mars can be thermodynamically stable (Zolotov, 1989; Zolotov et al., 1997) and the fact that the salt content in the Martian regolith may vary within 8–25 vol % (Clark and Van Hart, 1981). According to the data of telescopic spectral infrared observations (Pollack et al., 1990) and the existing measurements of the chemical composition of the Martian ground carried out by the Viking 1 and 2 (Banin et al., 1992) and Mars Pathfinder landers (Reider et al., 1997; McSween et al., 1999), the dominant salts on Mars are most likely to be represented by magnesium and calcium sulfates and chlorides MgSO4, CaSO4, MgCl2, and CaCl2. For instance, the measured sulfur and chlorine contents in the Martian ground can apparently correspond to 5 to 10% of sulfates and up to 1.5% of chlorides (Clark and Van Hart, 1981). Infrared telescopic observations of Mars (Pollack et al., 1990) are quite consistent with the indicated values of sulfate content. It was inferred from the analysis of these spectra that the observed spectral features at wavelengths of 8.7 and 9.7 μm agree well with the presence in the dust component of the Martian atmosphere of both sulfates and carbonates at content levels of 10–15 and 1–3%, respectively (Pollack et al., 1990). According to the data of spectral measurements carried out in the last few years by the Mars Global Surveyor thermal emission spectrometer TES, the cases in which the content of sulfates and carbonates (in the uppermost, 1–2-mmthick near-surface layer of the Martian ground) in amounts surpassing the TES detection limit, i.e., above 10–15% for sulfates and 5–10% for carbonates, have not been found. This, however, does not rule out their presence in amounts equal to and less than the above limit (Christensen et al., 2001). Taking into account the indicated contents of salts in the Martian ground, one can obtain a reasonable estimate for the water content bounded in hydrates of sulfates, chlorides and carbonates. To evaluate the possible content of bound water in the ground of circumpolar regions of Mars, we can assume that 15% (by volume) of all salts and carbonates in the Martian ground is represented mostly by sulfates (in proportion: 10% of magnesium sulfate and 5% of calcium sulfate), 2% by calcium chloride, and 5% by carbonates. As follows from the theoretical thermodynamic analysis (Zolotov et al., 1997), hydrates of sulfates and chlorides can exist in a stable state in the nearsurface ground at high latitudes of Mars (>45°) and contain 2 to 7 molecules in sulfates and 6 molecules in chlorides. When calculating the water content in carbonates, we considered hydrated carbonates of the monohydrocalcite (CaCO·H2O) and nesquehonite (MgCO3·3H2O) types. Therefore, knowing the molar fraction of water in the aforementioned water–bearing minerals and taking the indicated proportions of the content of salts in the Martian ground (with ground densities of 1.1 and 1.3 g/cm3), the possible volume fraction of bound water in a 1-m ground-surface layer (in the near-polar regions of Mars) can be estimated as about 8.6–10.5%. It is worthwhile noting that, along with water bound in salt hydrates, the Martian ground may also contain an additional amount of water bounded in layered silicates (e.g., in clay minerals of the montmorillonite group) and in products of the chemical weathering of basalts (in palagonite), which may constitute a few percent ground substance on Mars (Soderblom et al., 1992).
Since the quantitative relationships between weathering products and unweathered material in the Martian ground may vary from area to area, and the content of bound water (within high latitudes) may also notably change. Thus, using the above estimates of the potential content of bound water in the near-surface layer of Mars, we can also improve the estimates of water content in the underlying ground horizon (Boynton et al., 2002; Mitrofanov et al., 2002; Feldman et al., 2002), which have been obtained earlier using HEND measurement data. As a whole, the HEND data provide unique information about the properties of the volatilerich near-surface regolith layer. It is expected that these data will permit obtaining quantitative estimates of seasonal variations of the content of free (ice) and bound water in the ground at high latitudes of the planet.
This study was supported by the Russian Foundation for Basic Research (project no. 03-02-16644).
1. Banin, A., Clark, B.C., and Waenke, H., Surface Chemistry and Mineralogy, Mars, Kiefer, H.H., Ed., Univ. of Arizona Press, 1992, pp. 594–625.
2. Boynton, W.V., Feldman, W.C., Squyres, S.W., et al., Distribution of Hydrogen in the Near Surface of Mars: Evidence for Subsurface Ice Deposits, Science, 2002, vol. 297, pp. 81–87.
3. Christensen, P.R., Bandfield, J.L., Hamilton, V.C., et al., M., Mars Global Surveyor Thermal Emission Spectrometer Experiment: Investigation Description and Surface Science Results, J. Geophys. Res., 2001, vol. 106, pp. 23823–23871.
4. Clark, B.C. and Van Hart, D.C., The Salts of Mars, Icarus, 1981, vol. 45, pp. 370–378.
5. Clifford, S.M. and Hillel, D., The Stability of Ground Ice in the Equatorial Regions of Mars, J. Geophys. Res., 1983, vol. 88, pp. 2456–2474.
6. Clifford, S.V., Bartels, C.J., and Rubenstein, E.F., The Mars Thermal Model (MARSTHERM): A FORTRAN 77 Finite-Difference Program Designed for General Distribution, Houston: LPI, 1987.
7. Fanale, F.P., Martian Volatiles: Their Degassing History and Geochemical Fate, Icarus, 1976, vol. 28, pp. 179–202.
8. Fanale, F.P., The Water and Other Volatiles of Mars, Sci. Technol. Ser Am. Astronautical Soc. Publ., 1986, vol. 71, pp. 157–174.
9. Farmer, C.B. and Doms, P.E., Global and Seasonal Water Vapor on Mars and Implications for Permafrost, J. Geophys. Res., 1979, vol. 84, pp. 2881–2888.
10. Feldman, W.C., Boynton, W.V., and Drake, D.M., Planetary Neutron Spectroscopy from Orbit, in Remote Geochemical Analysis: Elemental and Mineralogical Composition, Piters, C.M. and Englert, A.J., Eds., Cambridge, Cambridge Univ. Press, 1993, pp. 213–234.
11. Feldman, W.C., Boynton, W.V., Tokar, R.L., et al., Global Distribution of Neutrons From Mars: Results From Mars Odyssey, Science, 2002, vol. 297, pp. 75–78.
12. Greeley, R. and Guest, J.E., Geologic Map of the Eastern Equatorial Region of Mars, US Geologic Survey, Misc. Inv., map I-1802-B, 1987.
13. Kuzmin, R.O., On the Structure of the Martian Cryolithosphere, in Problemy kriolitologii (Problems of Cryolithology), iss. 6, Moscow: Izd. MGU, 1977, pp. 7–23.
14. Kuzmin, R.O., Kriolitosfera Marsa (Martian Cryolithosphere), Moscow: Nauka, 1983.
15. Kuzmin, R.O., Bobina, N.N., Zabalueva, E.V., and Shashkina, V.P., Structural Inhomogeneities of the Martian Cryolithosphere, Astron. Vestn., 1988, vol. 22, no. 3, pp. 195–212.
16. Kuzmin, R.O., Komarov, I.A., Isaev, V.S., et al., The Comparative Morphometric Analysis of Polygonal Terrains on Mars and the Earth High Latitude Areas, Lunar Planet. Sci. Conf. XXXII, 2002, Abstract # 2030.
17. Kuzmin, R.O., Specific Character of the Superficial Layer of the Martian Cryolithosphere, Workshop HEND, 2002 May 20–22 (www.iki.rssi.ru/eng/hendws.htm).
18. Kuzmin, R.O., Mitrofanov, I.G., Litvak, M.L., et al., Mars: Detaching of the Free Water Signature (FTS) Presence Regions on the Base of HEND/Odyssey Data and Their Correlation with Some Permafrost Features from MOC Data, Lunar Planet. Sci. Conf. XXXIV, 2003, Abstract # 1369.
19. Kuzmin, R.O. and Zabalueva, E.V., Polygonal Terrains on Mars: Preliminary Results of Global Mapping of Their Spatial Distribution, Lunar Planet. Sci. Conf. XXXIV, 2003, Abstract # 1912.
20. Leighton, R.B. and Murray, B.C., Behavior of Carbon Dioxide and Other Volatiles on Mars, Science, 1966, vol. 153, pp. 136–144.
21. Litvak, M.L., Mitrofanov, I.G., Kozyrev, A.S., et al., Seasonal Variations of Subsurface Hydrogen as Seen by High Energy Neutron Detector, Mars Odyssey, Vernadsky-Brown Microsymposium no. 36, abstract no. 063, CD-ROM, Moscow, 2002.
22. McSween, H.Y., Murchie, S.L., Crisp, J.A., et al., Chemical, Multispectral, and Textural Constraints on the Composition and Origin of Rocks at the Mars Pathfinder Landing Site, J. Geophys. Res., 1999, vol. 104, pp. 8679–8716.
23. Mellon, M.T. and Jakosky, B.M., The Distribution and Behavior of Martian Ground Ice during Past and Present Epochs, J. Geophys. Res., 1995, vol. 100, pp. 11781–11800.
24. Mellon, M.T., Kretke, K.A., Smith, M.D., et al., A Global Map of Thermal Inertia from Mars Global Surveyor Mapping-Mission Data, Lunar Planet Sci. Conf. XXXIII, 2002, Abstract # 1416.
25. Mitrofanov, I., Anfimov, D., Kozyrev, A., et al., Maps of Subsurface Hydrogen From High Energy Neutron Detector, Science, 2002, vol. 297, pp. 78–81.
26. Pollack, J.B., Roush, T., Witteborn, F., et al., Thermal Emission Spectra of Mars (5.4–10.5 mm), J. Geophys. Res., 1990, vol. 95, pp. 14595–14627.
27. Reider, R., Economov, T., Wanke, H., et al., The Chemical Composition of Martian Soil and Rocks Returned by the Mobile X-Ray Mode, Science, 1997, vol. 278, pp. 1771–1774.
28. Romanovskii, N.N., Formirovanie poligonal'no-zhil'nykh struktur (Formation of Polygonal-veined Structures), Novosibirsk: Nauka, 1977.
29. Scott, D. and Tanaka, K., Geologic Map of the Western Equatorial Region of Mars, US Geological Survey, Misc. Inv.Map I-802-A, 1986.
30. Seibert, N.M. and Kargel, J.S., Small-Scale Martian Polygonal Terrain: Implications for Liquid Surface Water, Geophys. Res. Lett., 2001, vol. 28, no. 5, pp. 899–902.
31. Smith, D.E., Zuber, M.T., Frey, H., et al., The Global Topography of Mars and Implication for Surface Evolution, Science, 1999, vol. 284, pp. 1495–1503.
32. Smith, D.E., Zuber, M.T., and Neumann, G.A., Seasonal Variations of Snow Depth on Mars, Science, 2001, vol. 294, pp. 2141–2146.
33. Soderblom, L., The Composition and Mineralogy of the Martian Surface from Spectroscopic Observations: 0.3 μm–50 μm, in Mars, Kieffer, H.H., et al., Eds., Tucson: Univ. of Arizona Press, 1992, pp. 557–593.
34. Surkov, Yu.A., Shcheglov, O.P., Ryvkin, M.L., and Vinogradova, O.A., Neutron Spectrometry, in Remote Geochemical Analysis: Elemental and Mineralogical Composition, Piters, C.M. and Englert, A.J., Eds., Cambridge: Cambridge Univ. Press, 1998, pp. 427–442.
35. Yoshikawa, K., Contraction Cracking and Ice Wedge Polygons in Mars, Second Mars Polar Sci. Conf., 2000, Abstract # 4045.
36. Zolotov, M.Yu., Water-bearing Minerals in the Martian Soil (Thermodynamic Prediction of Stability), Lunar Planet, Sci. Conf. XX, 1989, pp. 1257–1258.
37. Zolotov, M.Yu., Zabalueva, E.V., and Kuzmin, R.O., Stability of Hydrated Salts and Goethite within the Desiccated Upper Layer of the Martian Regolith, Lunar Planet. Sci. Conf. XXVIII, 1997, pp. 1633–1634.
38. Zurek, R.W., Barnes, J.B., Haberle, R.M., et al., Dynamics of the Atmosphere of Mars, in Mars, Kieffer, H.H., et al., Eds., Tucson: Univ. of Arizona Press, 1992, pp. 835–933.