Seasonal Redistribution of Water in the Surficial Martian Regolith: Results from the Mars Odyssey High-Energy Neutron Detector (HEND)
R. O. Kuzmina, E. V. Zabaluevaa, I. G. Mitrofanovb, M. L. Litvakb, A. V. Rodinb, W. V. Boyntonc, and R. S. Saundersd
aVernadsky Institute of Geochemistry and Analytical Chemistry, Russian Academy of Sciences, ul. Kosygina 19, Moscow, 117975 Russia
bSpace Research Institute, Russian Academy of Sciences, ul. Profsoyuznaya 84/32, Moscow, 117810 Russia
cLunar and Planetary Laboratory, University of Arizona, Tucson, AZ 85721 USA
dNASA Headquarters, Washington, DC 20514 USA
Abstract - The seasonal variation of neutron emissions from Mars in different spectral intervals measured by the HEND neutron detector for the entire Martian year are analyzed. Based on these data, the spatial variations of the neutron emissions from the planet are globally mapped as a function of season, and the dynamics of seasonal variation of neutron fluxes with different energies is analyzed in detail. No differences were found between seasonal regimes of neutron fluxes in different energy ranges in the southern hemisphere of Mars, while the regime of fast neutrons (with higher energies) during the northern winter strongly differs from that during the southern winter. In winter ( Ls = 270°–330°), the fast neutron fluxes are noticeably reduced in the northern hemisphere (along with the consecutive thickening of the seasonal cap of solid carbon dioxide). This provides evidence of a temporary increase in the water content in the effective layer of neutron generation. According to the obtained estimates, the observed reduction of the flux of fast neutrons in the effective layer corresponds to an increase in the water abundance of up to 5% in the seasonal polar cap (70°–90°N), about 3% at mid-latitudes, and from 1.5 to 2% at low latitudes. The freezing out of atmospheric water at the planetary surface (at middle and high latitudes) and the hydration of salt minerals composing the Martian soil are considered as the main processes responsible for the temporary increase in the water content in the soil and upper layer of the seasonal polar cap. The meridional atmospheric transport of water vapor from the summer southern to the winter northern hemisphere within the Hadley circulation cell is a basic process that delivers water to the subsurface soil layer and ensures the observed scale of the seasonal increase in water abundance. In the summer northern hemisphere, the similar Hadley circulation cell transports mainly dry air masses to the winter southern hemisphere. The point is that the water vapor becomes saturated at lower heights during aphelion, and the bulk of the atmospheric water mass is captured in the near-equatorial cloudy belt and, thus, is only weakly transferred to the southern hemisphere. This phenomenon, known as the Clancy effect, was suggested by Clancy et al. (1996) as a basic mechanism for the explanation of the interhemispheric asymmetry of water storage in permanent polar caps. The asymmetry of seasonal meridional circulation of the Martian atmosphere seems to be another factor determining the asymmetry of the seasonal water redistribution in the "atmosphere–regolith–seasonal polar caps" system, found in the peculiarities of the seasonal regime of the neutron emission of Mars.
DOI: 10.1134/S0038094607020013 - http://www.springerlink.com/content/g1v12r57u6887360/
-------------------------------------------------------------------------------------------------------------------------------------------------
-------------------------------------------------------------------------------------------------------------------------------------------------The method of neutron spectroscopy, in recent years applied to the investigation of the Martian surface, provided the first estimates of the abundance of ground ice in the polar subsurface layer of the planetary cryolithosphere and of the depth of the ice at different latitudes (Boynton et al., 2002; Feldman et al., 2002; Mitrofanov et al., 2002, 2003, 2004). The mapping of neutron emission of Mars by the Russian neutron detector HEND and the American neutron spectrometer on board the Mars Odyssey spacecraft demonstrated that the surface regolith layer (up to 2 m thick) at high latitudes (higher than 60° ) of both hemispheres is characterized by the large abundance of hydrogen. The basic carriers of hydrogen in the Martian ground are molecules of water, which is mostly in the form of ice at high latitudes on Mars. Here, the mean volume content of ice exceeds 60 wt % at some points (Mitrofanov et al., 2003, 2004; Feldman et al., 2003). A comparison of the HEND data with model-predicted counts showed that the homogeneous soil model, in which the ground-ice abundances are high starting from the very surface, best agrees with the neutron emission data at high latitudes of the northern hemisphere of Mars (Mitrofanov et al., 2004). The mean water-equivalent contents in the subsurface soil layer, estimated using a homogeneous model in this polar region, were 44, 25, and 13 wt % to the north of 80° N and in the 70°-80° N and 60°–70° N latitude belts, respectively. A different situation was found in the southern polar region (to the south of 70° ), where the best agreement between the counts predicted by the model and the neutron emission measurements was obtained for the two-layer soil model, where the upper layer, composed of dry soil with a water content of up to 2 wt %, is from 10 to 20 cm thick and the lower layer is saturated with ice and characterized by 55 wt % water (Mitrofanov et al., 2004). Outside this region, in the 60°–70° S latitude belt, the water content in the lower layer decreases, averaging just 25%. For most of the Martian surface in the ±50° latitude belt, the estimated soil water contents vary from 1 to 10%. The revealed features of soil water content on Mars are based on neutron flux measurements obtained in wide energy ranges in both Martian hemispheres in summer, when the surface soil in the polar regions is not shielded from cosmic rays by the seasonal polar cap of CO2 snow. The distribution of spatial variation in an abundance of water in Martian soil corresponds to that in the subsurface regolith layer, whose thickness is comparable to that of the effective layer of generation of epithermal neutrons (1.5–2 m). However, the thermal regime of the subsurface soil layer strongly varies during the Martian year as seasons change (Haberle et al., 1993; Forget et al., 1999), and large variations in water vapor content are observed in the atmospheric column (Jakosky and Farmer, 1982; Smith, 2002, 2004; Fedorova et al., 2004a). The water transport from one hemisphere to another is also changed because of the seasonal reconstruction of the general circulation system of the Martian atmosphere (Clancy et al., 1996; Richardson and Wilson, 2002; Montmessin et al., 2004). In this context, how effective seasonal variations in the present-day Martian climate can be in inducing spatiotemporal variations of water content in the surficial regolith and what water contents result from this effect become important. One of the approaches to understanding this is to thoroughly analyze the seasonal variations of neutron fluxes from Mars in different energy ranges, corresponding to different effective layers of neutron generation. This was one of the goals of our study.
The HEND measurements of neutron emission from Mars cover an observation period of more than one Martian year (which is equal to two terrestrial years). This gave us the chance to study seasonal variations of the neutron emission from the Martian surface. To this end, the observations accumulated during the entire Martian year were partitioned into relatively small time intervals (15° Ls ). Since the neutron fluxes mainly depend on the hydrogen soil content (the higher the hydrogen content, the lower the neutron flux), we can try to explain the peculiarities of the seasonal redistribution of water in the "atmosphere-regolith" system and provide quantitative estimates of this redistribution based on the analysis of variations in the neutron emission from each specific surface area of Mars. The main goal of this study was to investigate the seasonal dynamics of neutron emission in different energy ranges coming from Mars during the entire Martian year. This dynamics is an indicator of the specific features of the seasonal redistribution of water, both in its solid phase and in its chemically-bound form, within the Martian ground.
Two groups of HEND measurements of neutron emission were applied in the study. The first group contains measurements of epithermal and fast neutron fluxes in two wide energy ranges, from 10 keV to 1 MeV and above 2.5 MeV, respectively. The second group is represented by the neutron flux data obtained in smaller energy ranges, namely, in two ranges for epithermal neutrons (100 eV–10 keV and 10 keV–1 MeV) and in two ranges for fast neutrons (1 MeV–2.5 MeV and 2.5 MeV–10 MeV). The effective layers of neutron generation corresponding to these ranges are ~1.5–2 m, ~1 m, ~0.4–0.5 m, and ~0.2–0.3 m, respectively. Both the measured HEND counts and the normalized neutron fluxes at the height of the Mars Odyssey orbit were used in the analysis of neutron emission. The flux of cosmic rays that reaches the Martian surface is the main factor behind neutron generation in the subsurface layer of the planet. However, this flux can vary in time by a factor of several for a number of reasons (Mitrofanov et al., 2004). Of course, this makes the interpretation of experimental data more difficult when we consider naturals seasonal variations of the neutron flux from the surface layer of Mars. To avoid such errors, the measurements were normalized (individually for each season) by the neutron flux from the Solis Planum region, which is the brightest area of neutron emission and, hence, the driest area on Mars ( 16°–53° S, 63°–114° W). The neutron emission from this region is well described by the homogeneous soil model with a uniform depth distribution of moisture and the element composition corresponding to the composition of the Martian soil obtained by Mars Pathfinder at its landing site in Chryse Planitia (Mitrofanov et al., 2004). The normalization of the neutron flux carried out earlier in the experiment with the neutron spectrometer (normalization to the layer of the seasonal polar cap composed of 100% solid carbon dioxide) and in the analysis of the measurements of gamma emission from the Martian surface (normalization to the layer of the permanent cap of 100% water ice in summer) demonstrated that the soil water content in the Solis Planum region is 2 mass % and only changes slightly from season to season (Boynton et al., 2003; Feldman et al., 2003). The normalization of the measured neutron fluxes on Mars to the neutron emission from the Solis Planum region allowed us to perform a more correct comparison of the variations in relative and absolute (modeled) water contents in the Martian soil in different regions of the planet.
The Character of the Frequency Distribution of Neutron Emission of Mars
Previously, it has been demonstrated (Mitrofanov et al., 2003; Litvak et al., 2003; Kuzmin et al., 2004b) that Martian surface regions with low neutron emission are mainly located in the polar zone of the planet (poleward of 60° ). Equatorward of this latitude, up to ~ 50° in both hemispheres, the largest gradient of neutron flux change (from the lowest to highest values) is observed. High values are typical for the majority of the Martian surface in the ±50° latitude belt. Statistical analysis of spatial variations of neutron emission from Mars in the northern summer (the influence of the seasonal polar caps of solid carbon dioxide was not taken into account) demonstrated that the frequency distribution of the normalized epithermal and fast neutron fluxes is clearly bimodal (Fig. 1). Figures 1a and 1b show a relatively sharp change from low to high neutron fluxes at 0.45 for epithermal neutrons and at about 0.7 for fast neutrons. It is obvious (Figs. 1c and 1d) that low-flux populations dominate for both epithermal (the average flux is 0.3) and fast neutrons (the average is 0.65) in the polar regions of Mars. This frequency distribution indicates that the Martian neutron emission in different energy ranges is composed of two populations of neutron fluxes (low energy and high energy), which are rather strictly separated in space.
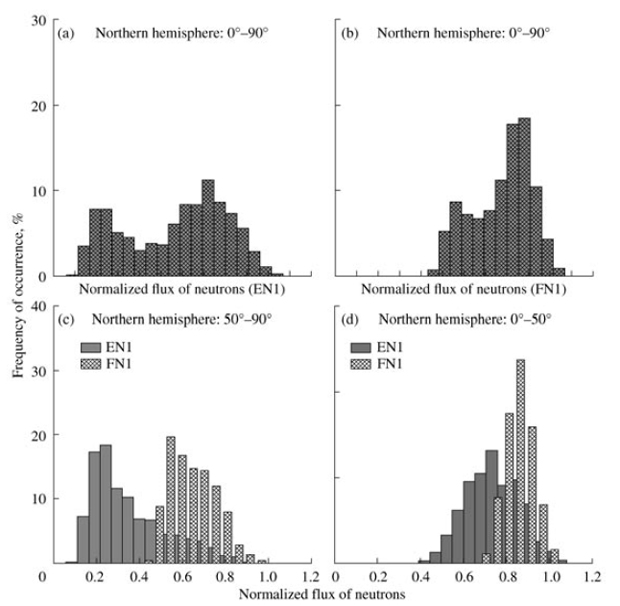
Fig. 1. The frequency distribution of normalized fluxes of epithermal (EN1) and fast (FN1) neutrons in the northern hemisphere of Mars (a, b) and at high latitudes (c, d).
In the present climate of Mars, the stable ground ice roof lies at very different depths in the Martian regolith depending on geographical latitude (Farmer and Doms, 1979). For most of the planet (in the ±50° latitude belt), the ice can be stable only at depths that are several times larger than the thickness of the effective layers of generation of neutrons measured by the HEND instrument (1.5–2 m). Numerical computations (Mellon et al., 1995, 2004) show that the depth of the top of stable ground-ice masses can vary from tens of centimeters to 1 m at latitudes higher than 60° and from 1 m to several meters as latitude decreases to 50°. According to the measurements of the HEND neutron spectrometer, it is at the 60° latitude (in both hemispheres) that an abrupt change from high to low contents of soil water occurs (Mitrofanov et al., 2003); the water-equivalent abundance decreases rather fast from 15–20% to 5% at latitudes from 60° to 50° . This latitude belt seems to be a transitional zone from the region with neutron fluxes of one population to the region with fluxes of the other population. In this transitional zone, soil water can simultaneously be in the form of ice and in the chemically bound form in the structure of hydrated minerals. According to estimates by Kuzmin et al. (2004b), the maximal abundance of chemically bound water in a meter soil layer at high latitudes of Mars can be 8.6-10.5%. At lower latitudes, the fraction of ground ice in the surficial regolith gradually decreases due to the lowering of its top level (Mellon et al., 2004), and the chemically bound water becomes a dominating hydrogen carrier (Kuzmin et al., 2004b). Since at latitudes where the top of the stable ground ice is at depths less than the thickness of the effective layer of the generation of fast neutrons (0.1–0.3 m), the population of low neutron fluxes is a reliable indicator that masses of free water (in the form of ground ice) are the dominating carriers of high hydrogen concentrations in the Martian soil. The direct correlation between the location of polygonal terrains affected by frost cracking (the basic morphologic indicator of ice-rich geological materials on Earth) with regions with low neutron fluxes, recently found by Kuzmin and Zabalueva (2003), is convincing evidence that the population of low neutron fluxes actually characterizes regions of high ground-ice concentrations. In areas where ice can be stable only at depths exceeding the thickness of the effective layer of the generation of epithermal neutrons (1.5–2 m), the population of high fluxes is indicative of the presence of some other carrier of hydrogen in the soil, which occupies a smaller volume. Physically and chemically bound water (adsorbed and contained in crystalline hydrates of salts, respectively) seems to act as such a carrier.
Seasonal Variations in the Neutron Emission of Mars
There are several processes under Martian conditions that are reversible in time and can directly affect the redistribution of water in the "atmosphere-regolith–polar caps" system. These are adsorption/desorption of water vapor in the ground, hydration/dehydration of salt minerals in the Martian regolith, and condensation/sublimation of both frost and ground ice in the regolith and water ice on the surface of the polar caps (Fig. 2). The intensity of these processes is strongly dependent on such time-variable climatic parameters as atmospheric and surface temperature, water vapor content in the Martian atmosphere, and specific features of atmospheric seasonal circulation (Tokano, 2005; Kuzmin, 2005). Since the above listed processes manifest themselves on the ground surface and at depth in different ways in different seasons (both in depth and in space), the analysis of seasonal variations of neutron emission helps us to understand which process is most important for the cycle of water exchange between the atmosphere and the regolith.
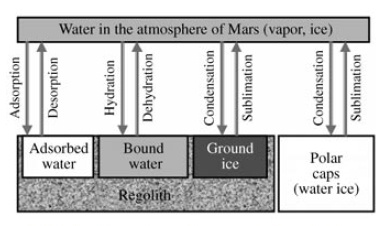
Fig. 2. The scheme of reversible processes taking part in the water cycle on Mars in the "atmosphere-regolith–polar caps" system and the forms and phases of water in this system.
Our study of seasonal variations of the neutron emission of Mars was based on the model relation between the energy of measured neutron fluxes and the most probable thicknesses of effective layers of waterbearing ground, where neutrons are generated. Thus, for example, the rate of generation of fast neutrons (with energies above 1 MeV) is maximum at depths of about several tens of centimeters, while for epithermal neutrons (with energies less than 1 MeV) this maximum is at a depth of 1.5–2 m (Mitrofanov et al., 2003). An important advantage of the HEND neutron detector, as compared to the American neutron spectrometer, is its ability to measure the neutron emission from Mars in a much wider energy range (from 0.4 eV to 15 MeV) due to the presence of several independent detectors. The measured data on neutron fluxes in such a wide energy range can be partitioned into smaller energy subranges. Figure 3 presents a comparison of the effective layers of neutron generation in different energy ranges in the polar regions of Mars in summer and winter. Such a partition of neutron flux measurements made it possible to reconstruct the character of water distribution both in space and in depth from layer to layer (within 2 m) as a function of Martian seasons.
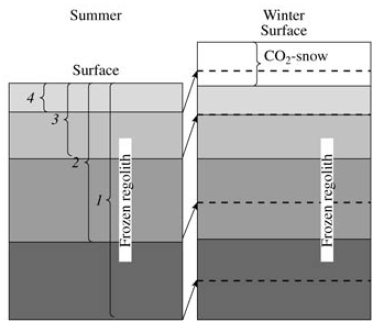
Fig. 3. Comparison of the effective layers of neutron generation in different energy ranges in the polar regions of Mars in summer and winter: (1) is the effective layer of generation of epithermal neutrons with energies from 100 eV to 10 keV (~1.5–2 m); (2) is the effective layer of generation of epithermal neutrons with energies from 10 keV to 1 MeV (~1m); (3) is the effective layer of generation of fast neutrons with energies from 1 to 2.5 MeV (~0.4-0.5 m); (4) is the effective layer of generation of fast neutrons with energies from 2.5 to 10 MeV (~0.2–0.3 m).
The first results of the global mapping of neutron emission of Mars (Mitrofanov et al., 2003; Litvak et al., 2003) demonstrated that the main tendency in the seasonal variations of neutron fluxes at high latitudes of Mars is clearly determined by the seasonal behavior of the processes of condensation/sublimation of solid carbon dioxide within the seasonal polar caps. The cover of solid carbon dioxide usually isolates the lower icerich ground from the influence of cosmic radiation. Starting from autumn, the neutron fluxes gradually grow as the cap becomes thicker and reach their maximum values when the thickness of the solid carbon dioxide cover is maximum (Litvak et al., 2003, 2006). However, in summer (when there are no seasonal caps), the neutron fluxes in the polar regions are minimum because the neutrons actively interact with hydrogen atoms directly within the ice-rich ground. This tendency is obvious for epithermal neutrons in both Martian hemispheres as well as for fast neutrons, but only in the southern hemisphere. The results of the global mapping of fast neutron fluxes for various seasons (Kuzmin et al., 2005) conclusively demonstrated that the seasonal regime of fast neutrons is much different from that of epithermal neutrons in the northern hemisphere. This difference is most clear for northern winter. Contrary to epithermal neutrons, low fluxes of fast neutrons persist in autumn–winter (when the seasonal cap of CO2 snow grows) not only within the growing seasonal cap, but also far beyond it. The analysis of the seasonal behavior of the neutron fluxes in different energy ranges demonstrated a considerable reduction of fast neutron fluxes in the first half of winter ( Ls = 270°–330° ) (Fig. 4). The general autumn–winter positive trend in the epithermal neutron flux was complemented during this season by "a deflection" in the reduction of high-energy neutron fluxes. The following tendency is clearly seen: the higher the energy range of neutrons, the stronger "the deflection" in the flux reduction. To investigate the spatial scale of this phenomenon and its relation to the thickness of the effective layer of neutron generation, we analyzed seasonal variations of the neutron emission of Mars separately for each of the four energy ranges under consideration: two for epithermal neutrons (EN1 and EN2) and two for fast neutrons (FN1 and FN2). The neutron emission measured in these energy ranges was analyzed as a function of the areocentric longitude of the Sun (indicating the season) and geographic latitude. The values were first normalized to the neutron flux from the driest area of the planet, Solis Planum, where the soil water content is less than 2% (Mitrofanov et al., 2003). To globally map the seasonal dynamics of neutron fluxes, the measurements were averaged over a 10° latitude belt and a 15° interval of areocentric longitudes of the Sun ( Ls ) for each energy range. According to the resulting maps, showing the seasonal dynamics of the neutron fluxes from the Martian surface (Fig. 5), the regimes of epithermal and fast neutrons in the polar regions of the southern hemisphere (in all seasons) are characterized by a similar cyclicity, which directly depends on the seasonal behavior of condensation and sublimation of the CO2 snow cover in the seasonal polar cap. However, the neutron fluxes in the higher energy ranges (EN2, FN1, and FN2) show quite a different pattern of seasonal dynamics in the northern hemisphere of the planet. This abnormal seasonal regime of neutron emission in the northern hemisphere was manifested in the maps of seasonal dynamics as two clearly pronounced meridionally aligned "troughs" of low fluxes of highenergy neutrons (see Fig. 5). The less deep and short "trough" was observed during the northern summer ( Ls = 140°–170° ) and could be seen from the mid-latitudes to the equator. The second larger "trough" appeared in the first half of the northern winter ( Ls = 270°–330° ) and extended from the polar region to low latitudes of the southern hemisphere. This winter "trough" is characterized by the stronger reduction of neutron fluxes as compared to the summer "trough." Note that the sublimating residual polar caps of Mars were a basic source of water in the Martian atmosphere during the periods when both "troughs" appeared. It can be seen from Fig. 5 that the winter decrease in the flux of high-energy neutrons was observed in a wide latitude range and occurred even against the background of the thickening of the seasonal cap of solid carbon dioxide in the polar region. The flux of highenergy neutrons demonstrated the greatest fall in the period Ls = 270°-320° (by 14–17% in the 50°-60° latitude belt, while the measurement error was about 10%). In addition, the reduction of the neutron flux decreased gradually when we passed from the thickest effective layer of neutron generation (EN2) to the thinnest (FN2). This means that the value of increase in water content consistently grew with decreasing depth within the meter layer. The relative change of the decrease in the fast neutron flux (with an effective layer of their generation of ~0.2-0.3 m) along the second "trough" from the pole to midlatitudes is shown in Fig. 6. This figure also demonstrates that the spread of the reduction (averaged over 10° latitude belts) in the fast neutron flux at latitudes from 10° N to 90° N was 7–9.5% of the flux at the start of its reduction (at Ls = 270° ). Note that the reduction of the fast neutron flux was somewhat higher at the periphery of the seasonal polar cap ( 60°– 70° N) than in its central part during the period Ls = 270°–330° . A slight increase in the reduction of the fast neutron flux was also observed in the 20°–30° N latitude belt, where, according to data from the TES thermal emission spectrometer onboard the Mars Global Surveyor , the second seasonal maximum of the atmospheric water content is observed during the northern winter (Smith, 2002, 2004). To estimate the possible increment of water content corresponding to the observed reduction of the neutron flux, we used a numerical simulation method similar to that developed to convert the measured neutron fluxes to the equivalent water contents (Mitrofanov et al., 2004; Litvak et al., 2006). According to our estimates (see Fig. 6), the observed reduction of the fast neutron flux corresponds to an increase in the water content of up to 5% within the seasonal polar cap ( 70°–90°N), about 3% at middle latitudes, and 1.5–2% at low latitudes. Thus, the soil water content demonstrates a distinct tendency of growing from low latitudes to the polar region. The analysis of the seasonal dynamics of neutron fluxes measured by the HEND instrument shows that a considerable redistribution of water takes place in the uppermost surface regolith layer of the northern hemisphere of Mars and the measurements of fast neutron fluxes are most sensitive to the seasonal variations of water content in the Martian soil.
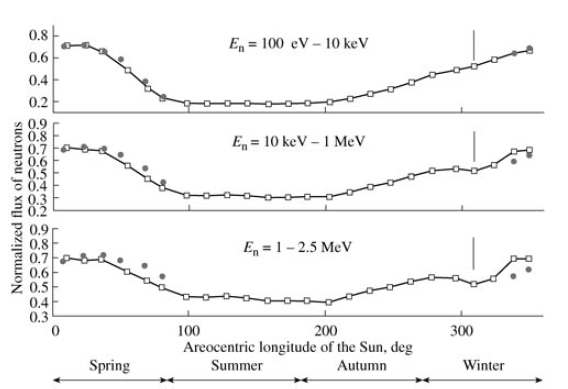
Fig. 4. The normalized flux of neutrons in different energy ranges as a function of the areocentric longitude of the Sun (Ls). The dependences are for the northern polar region (80°–90°N). The squares show measurements during the first total Martian year, while the circles are for measurements during the examined part of the second year. The vertical arrows indicate a seasonal reduction of the neutron flux.
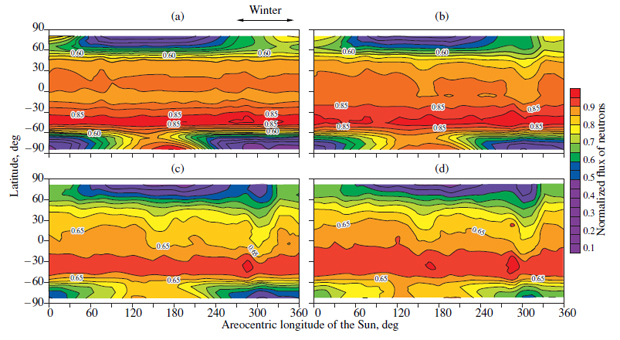
Fig. 5. Global maps of the seasonal dynamics of normalized fluxes of the neutron emission of Mars in different energy ranges: EN1 (a), EN2 (b), FN1 (c), and FN2 (d). The northern hemisphere winter is indicated by the double arrow.
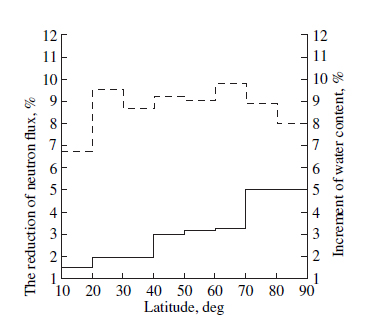
Fig. 6. The reduction of the normalized fluxes of fast neutrons with energies from 2.5 to 10 MeV (dashed line) and the increment of water content in the effective layer of generation of these neutrons (solid line) during the period from Ls = 270° to Ls = 30° as a function of latitude.
Fast Neutron Flux Variations in the Day–Night Cycle
To examine the character of diurnal variations of the neutron emission of Mars, we separately analyzed the HEND data for night and daysides of Mars (within the ±50° latitude belt) obtained in the northern hemisphere spring (Ls = 30°-60°). The interaction between the Martian atmosphere and the surface regolith for such a short time scale as a day occurs in a soil layer comparable to the skin layer of diurnal temperature oscillations. Depending on the thermal inertia of the surface material, the skin layer can have a thickness from ten to several tens of centimeters (Christensen and Moore, 1992). Therefore, in our analysis we used the fast neutron fluxes corresponding to a rather thin effective layer of their generation of about 20–30 cm. The results of global mapping of daytime and nighttime variations of the flux of fast neutrons are shown in Fig. 7a. The comparison of daytime and nighttime fluxes of neutrons reveals a considerable difference in their maps. These observations, if zonally averaged over 10° latitude intervals, clearly show that the fast neutron flux decreases to some extent at night, and this decrease is noticeably larger in the southern hemisphere than in the northern one during this season. The decrease in the nighttime flux of fast neutrons (with respect to the daytime flux) is 10–11% in the 40°-50° S latitude band, 5-6% in the equatorial zone, and 3–4% in the 40°-50° N latitude belt (see Fig. 7b). The model estimation of the reduction in the nighttime flux of neutrons and of the water equivalent by the method described in Mitrofanov et al. (2004) gives 0.5–1.5% for the increase in the soil water content at night. Since nighttime surface temperatures can reach values below the point of condensation of atmospheric moisture, and the relative humidity of the atmosphere is close to 100% at night, it is highly likely that the observed increase in the soil water content is caused by the sum effect of the following processes: the adsorption of water vapor in the ground, the frost formation on the surface and in the uppermost subsurface soil layer, and the hydration of salt minerals contained in the ground. Experimental and theoretical studies (Zent and Quinn, 1995; Zent et al., 2001; Tokano, 2003) show that the Martian ground is characterized by a rather low adsorptivity and contains about 0.1–0.2 mass % of adsorbed water. Therefore, it is unlikely that the adsorption/desorption processes could be responsible for the observed diurnal variations of the content of water in the Martian ground. It seems more likely that hydration affects diurnal variations of water content, if the ground contains salts, whose hydration rates are high and ensure the bounding of water molecules in the structure of salt minerals in a time frame comparable to the length of night. We also cannot exclude that mirabillite, a crystalline hydrate of sodium sulfate Na2SO4 • 10 H2O, could prove to be the most probable candidate for such salt in the Martian ground (Kuzmin et al., 2004b). The hydration rate of mirabilite is much higher than that of other sulfates and chlorides under Martian conditions. Hence, the processes of water condensation (the appearance of frost) and hydration of salts (the increase in the content of bound water) occurring at night can be considered as the most probable causes of the diurnal variations observed in the flux of fast neutrons. However, it is still impossible to estimate the individual contribution of each of these processes.
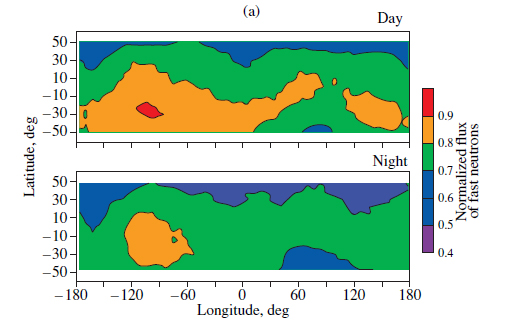
Fig. 7a. The observed differences of day and night measurements of normalized fluxes of fast neutrons in the ±50° latitude belt in spring (Ls = 30°–60°): (a) maps of spatial variations of normalized fluxes of fast neutrons (with an effective layer of their generation of 20–30 cm) for daytime (upper) and nighttime (bottom) on Mars.
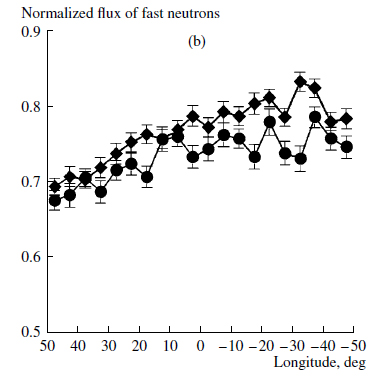
Fig. 7b. The observed differences of day and night measurements of normalized fluxes of fast neutrons in the ±50° latitude belt in spring (Ls = 30°–60°): (b) zonally averaged (over 10° latitude intervals) daytime (diamonds) and nighttime (circles) normalized fluxes of fast neutrons as a function of latitudes. Vertical bars show the error of the mean.
The efficiency of deceleration of fast neutrons in the subsurface layer of the planet depends on the presence of hydrogen atoms in the ground. If the ground contains even 1 mass % of hydrogen, the efficiency of neutron deceleration increases and the fast neutron flux coming out of the soil is approximately 10% less (Drake et al., 1988). Therefore, the temporary increase in the hydrogen concentration in the subsurface soil layer within the seasonal cap, resulting from an increase in the water content in this layer, can be considered as the most reasonable explanation of the winter decrease in the fast neutron flux on Mars. It has been mentioned above that the increase in the soil water content can be associated with the freezing out of atmospheric water into the ground, hydration of salt minerals, and the formation of carbon dioxide gas hydrate ( CO2 • 6H2O ) within the seasonal polar cap. However, substantial amounts of water should be delivered to the northern hemisphere in winter to make the influence of these processes noticeable. The observed reduction of the fast neutron flux in the period Ls = 270°–330° corresponds to a considerable increase in the water content in the ground (from 5% in the seasonal cap and 1.5–3% beyond the cap). This increase could not only be associated with the freezing out of small amounts of atmospheric water (10–15 precipitable microns) typically observed in the northern hemisphere in winter (Smith, 2002). What process could provide enough water for such a large seasonal increase in the water content in the subsurface layer? The meridional atmospheric transport of water vapor from the summer southern hemisphere at the moment when the polar cap is most rapidly subliming is the most realistic cause of moisture delivery to the northern hemisphere in winter. It is well known that the Hadley circulation cell is one of the basic mechanisms of the meridional transport of the atmospheric moisture from the southern to northern hemisphere of Mars (Zurek et al., 1992; Haberle et al., 1993; Clancy et al., 1996). This mechanism of meridional circulation is six times stronger on Mars than on Earth (Tokano, 2003). In particular, this mechanism is most efficiently manifested in the southern hemisphere in summer, when the intensity of the Hadley circulation cell, crossing the equator, is twice as high as in the northern summer. Seasonal differences in the height at which water vapor becomes saturated in the atmosphere of Mars can be considered as one of the main causes of such an asymmetric pattern of meridional circulation (Clancy et al., 1996; Richardson et al., 2002; Montmessin et al., 2004). Because of this, the summer clouds, formed in the upward branch of the Hadley cell, are at different heights in the northern and southern hemispheres. Figure 8 illustrates the Clancy effect (Clancy et al., 1996) for meridional water transport in the Martian atmosphere as simulated by the model of the general atmospheric circulation of Mars (Montmessin et al., 2004; Rodin and Wilson, 2006). In the present epoch, the moment when Mars passes its aphelion (the point of its orbit at which Mars is furthest from the Sun) and the flux of solar radiation and, hence, the surface and atmospheric temperatures of the planet are minimum, corresponds to the northern summer. Because of lower atmospheric temperatures and saturation altitudes of water vapor, a global belt of clouds, preventing the water vapor transfer from the northern to the southern hemisphere (see Fig. 8), is formed in this season in the ascending branch of the Hadley circulation cell (at low and middle latitudes of Mars). At the moment of perihelion (the closest position of Mars to the Sun), which coincides with summer in the southern hemisphere of Mars, the surface and atmospheric temperatures are considerably higher, and the saturation altitude of water vapor is almost twice as high as during aphelion. Therefore, no cloud "plug" is formed in this season and the entire atmospheric water vapor is glibly transferred to the northern hemisphere. It is the low position of the altitude of water vapor saturation at the moment of aphelion and, consequently, the formation of the global cloud belt that result in a strong orbital asymmetry of the meridional water vapor transport in the Martian atmosphere (Clancy et al., 1996). Rather large water masses are transferred to the northern hemisphere during one revolution of the Martian perihelion (51000 years). This explains the morphologic differ ence between the polar caps. Such a seasonal "pump" obviously changes its direction with periods of the perihelion revolution and precession of the rotation axis of Mars (with a period of 173000 years). A combined effect of the periods of the perihelion revolution and precession results in a cyclic change of the summer location of the Martian polar caps at perihelion. This explains the observed time asymmetry of the polar caps, whose age is about 25000 years. The alternative explanation of the asymmetry of the meridional transport of the atmospheric water, suggested by Richardson and Wilson (2002), is based on the global asymmetry of the Martian topography (lowlands in the northern hemisphere and highlands in the southern one). The meridional flow of the Hadley cell is directed to higher geopotentials during northern summer. This weakens the cell's intensity and, hence, decreases the meridional water transport promoting water storage mainly in the northern hemisphere. In the southern summer, on the contrary, the atmospheric flow "slithers" to low geopotentials and is more intense. Therefore, the meridional transport is also stronger, and water is efficiently transported to the northern hemisphere rather than stored in the southern one. However, the meridional water transport by atmospheric circulation cannot be considered as a one-dimensional stationary process. Because of very strong diurnal variations of temperature and a considerable tidal wind, which also demonstrates diurnal and semidiurnal periodicities, the condensed water precipitates onto the surface and then sublimates with a period of one day, that is, during the time when the air masses demonstrate only a negligible drift. Therefore, the seasonal migration of water on Mars should be considered as a general trend against the background of the fast quasi-periodic reversible process. According to numerical results of atmospheric general circulation models (with different factors of atmospheric water transfer taken into account), considerable amounts of condensed water (as compared to those in the atmospheric column), up to several centimeters and even 10 cm in some regions, can be accumulated on the surface in the northern hemisphere during the season. At the same time, a simple estimation taking into account only the mean meridional transport in the upward branch of the Hadley cell (from the southern hemisphere to the northern one) gives at least an order of magnitude smaller thicknesses of condensed water (up to 10 mm) poleward of 30° N. Regardless of the available theoretical estimates, the panoramic images of Utopia Planitia (Fig. 9) obtained from the Viking-2 lander on May 18, 1978, at 47.7° N during the northern winter (Ls = 310°) offer observable confirmation of the reality of the water condensation process on the Martian surface in the northern winter (in regions adjacent to the periphery of the seasonal polar cap). It can be seen from Fig. 10 that the surface was covered by a thin layer of water frost from 1 to 2 mm thick at the landing site of Viking-2 (Jones et al., 1979). As demonstrated above (see Fig. 9), the relative winter increase in water content in the subsurface layer of Mars (outside the seasonal cap), estimated from seasonal variations of fast neutron fluxes, is from 2 to 3 wt % in the ground layer of about 10 cm thick. If this water content is presented as precipitable water, we obtain 2–3 mm. By the order of magnitude, this estimate closely agrees both with the winter precipitable water calculated by a model of the mean meridional transport of atmospheric water in the upward branch of the Hadley cell and with the observed thickness of the frost layer at the landing site of Viking-2 . However, in addition to the freezing out of atmospheric water, the process of hydration of salt minerals, composing the Martian soil, can also considerably contribute to the observed increase in the water abundance in the surface regolith at latitudes lower than 50° N (outside the northern seasonal polar cap). According to measurements of the chemical composition of the Martian soil from several regions of Mars, the abundance of salt minerals in the Martian ground (in the form of sulfates and chlorides) can vary locally from 15 to 40% (Clark and Hart, 1981; Rieder et al., 2004; Squyres et al., 2004). When salts at such high concentrations are hydrated, the content of bound water in the Martian ground can become compatible (if not higher) to the amount of water condensed in winter on the surface of Mars. We cannot exclude that the formation of the trough of low fluxes of fast neutrons observed during the northern summer (at middle and low latitudes) is probably associated with the hydration of salt minerals. The seasonal maximum of water is at middle and low latitudes during this season, Ls = 150°–170° (see Smith, 2002), which favors the hydration process in the subsurface layer of the Martian ground. In addition, the destruction of the tropical cloud system (Clancy et al., 1996) and the reconstruction of zonal waves (Fedorova et al., 2004b) occur during this period, and this enhances the meridional north-to-south transport of atmospheric water for a short while. The results of a recent global mapping of the bound water in the surface regolith of Mars (Kuzmin et al., 2004a, 2006), performed by the Thermal Emission Spectrometer (TES) onboard the Mars Global Surveyor spacecraft, demonstrated a seasonal increase in the spectral index of chemically bound water at middle and low latitudes of the northern hemisphere (Fig. 10) during the period of formation of the winter trough of low fluxes of fast neutrons ( Ls = 270°–330° ). The observed increase in the bound water index is a direct indication of the enhancement of the hydration of salt minerals at middle and low latitudes in winter. Such a good time coincidence of the appearance of the trough of low fluxes of fast neutrons and the seasonal increase in the spectral index of bound water is a strong argument in favor of the considerable of the process of the hydration of salt minerals in the seasonal water redistribution within the surface regolith. We cannot rule out that the winter increase in the soil water content (1.5–3 wt %) at 10°– 50° N, obtained from the HEND data, in the first approximation can be considered a quantitative measure of the contribution introduced by the chemically bound water to the total balance of the seasonal water redistribution in the Martian regolith.
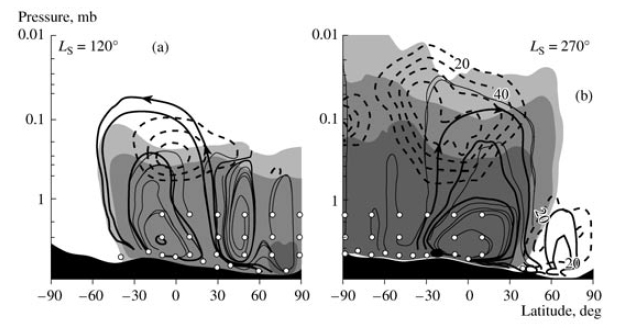
Fig. 8. Cross sections of the meridional water transport in the atmosphere of Mars at aphelion ((a) northern summer) and perihelion ((b) southern summer) constructed by numerical simulations in (Rodin and Wilson, 2006). The regions where water vapor is present in the Martian atmosphere are colored gray; the solid thick lines show the stream lines of the Hadley circulation cell; the thick arrows indicate the direction of the air mass displacement; and the thin lines show the meridional water advection in the Martian atmosphere according to the results of the atmospheric general circulation model taking into account phase transformations and cloud formation. The thick dashed lines show the localization of ice clouds, the corresponding cloud ice content is given in ppmv; and small circles schematically represent the sources of water vapor.
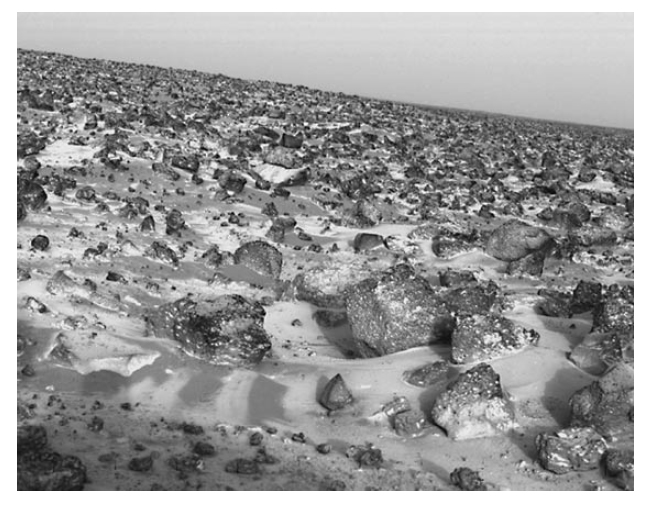
Fig. 9. Image of the thin cover of water frost (or snow) from 1 to 2 mm thick on the surface of Mars taken at the landing site of the Viking-2 lander (Utopia Planitia, 47.9° N, 224° W) in northern winter ( Ls = 310° ). The image PIA00533 from the NASA Planetary Photojournal taken on May 18, 1978, by a camera on Viking-2
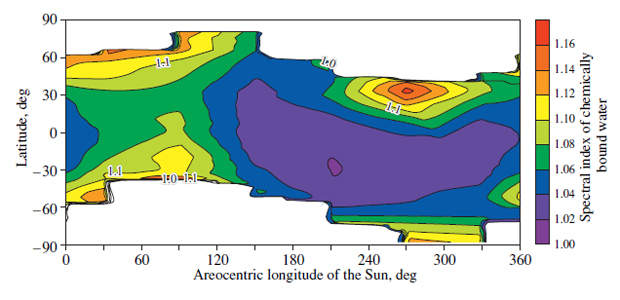
Fig. 10. The global map of the dynamics of seasonal variations of the bound water index in the surface regolith of Mars obtained from IR imaging of the Martian surface by the thermal emission spectrometer (TES) onboard the Mars Global Surveyor spacecraft for three Martian years. The bound water index is estimated from the intensity of the emission peak of bound water at a wavelength of 6.1 μm. According to Kuzmin et al. (2006).
The observed specific features of the seasonal variations of the neutron emission of Mars allow us to conclude that the seasonal water redistribution in the surface layer of the planet is clearly asymmetric with a considerable winter increase in the water abundance in the subsurface layer in the northern hemisphere with no similar effect in the southern hemisphere during this season. This character of the seasonal water redistribution in the surface regolith agrees with an asymmetric pattern of the structure of the Martian surface regolith, recently discovered by Mitrofanov et al. (2003, 2004) from HEND results; namely, the Martian regolith can be considered an ice-rich layer from the very surface in the northern hemisphere and as a two-layer structure (with a water-depleted upper layer) in the southern hemisphere. The revealed patterns of differences between the structures of the Martian regolith in two hemispheres of the planet and the peculiarities of seasonal redistribution of water in the northern hemisphere is reliable evidence that the southern hemisphere of Mars is really characterized by a negative water balance at the present position of its perihelion. The water balance in the northern hemisphere is positive because of the dominating transport of the atmospheric water from the summer southern hemisphere to the winter northern hemisphere. It should be noted that this tendency can be stronger or weaker depending on the character of the annual energy balance in the south polar cap region. The point is that in summer the water vapor entering the atmosphere in the southern hemisphere originates from sublimation of water ice deposits in the permanent polar cap, and these deposits are nonuniformly exposed due to shielding by the residual layer of solid carbon dioxide. Both the amount of atmospheric water vapor in the southern hemisphere and the potential volume of moisture transferred to the northern hemisphere during summer depend on the intensity of the water ice sublimation in the cap. However, variations of atmospheric water content in the southern hemisphere of the planet obtained both from surface-based (Jakosky and Barker, 1984; Clancy et al., 1992) and space-borne observations (Titus et al., 2002; Bibring et al., 2005) satisfy the idea that even in the modern climatic epoch the south residual polar cap can be in two stable states (Jakosky and Haberle, 1990; Jakosky et al., 2005). The first state is when a rather thick residual layer of carbon dioxide ice persists in summer and prevents sublimation of the water-ice deposits underneath. The second state is associated with maximal thinning of the carbon dioxide ice layer in summer, which promotes sublimation of water ice. Thus, depending on which of these states has been established, the intensity of water ice sublimation changes, and this determines the content of atmospheric water vapor in the southern hemisphere. Hence, the scale of the process of water redistribution in the regolith of the northern hemisphere in certain periods can also change depending on the stability of the residual CO2 ice cover in the south polar cap in summer.
This work was supported by the Russian Foundation for Basic Research, project no. 03-02-16644. A.V. Rodin acknowledges the support of the Russian Foundation for Basic Research (project no. 04-02-16856).
1. Bibring, J-P., Langevin, Y., Gendrin, A., et al., and the OMEGA Team, Mars Surface Diversity as Revealed by the OMEGA/ Mars Express Observations, Science, 2005, vol. 307, pp. 1576–1581.
2. Boynton, W.V., Feldman, W.C., Squyres, S.W., et al., Distribution of Hydrogen in the Near Surface of Mars: Evidence for Subsurface Ice Deposits, Science, 2002, vol. 297, pp. 81-85.
3. Boynton, W.V., Chamberlain, M., Feldman, M., et al., Abundance and Distribution of Ice in the Polar Regions of Mars: More Evidence for Wet Periods in the Recent Past, Proc. Sixth Int. Conf. on Mars, 2003, Abstract #3259.
4. Christensen, P.R. and Moore, H.J., The Martian Surface Layer, Mars , Kieffer, H.H., Jakosky, B.M., Snyder, C.W., et al., Eds., Tucson: Univ. Arizona Press, 1992, pp. 686–729.
5. Clancy, R.T., Grossman, A.W., and Muhleman, D.O., Mapping Mars Atmospheric Water Emission on 1.35 cm with the VLA, Icarus, 1992, vol. 100, no. 1, pp. 48–59.
6. Clancy, R.T., Grossman, A.W., Wolff, M.J., et al., Water Vapor Saturation at Low Latitudes Around Aphelion: A Key to Mars Climate?, Icarus , 1996, vol. 122, no. 1, pp. 36–62.
7. Clark, B.C. and van Hart, D.C., The Salts of Mars, Icarus , 1981, vol. 45, no. 3, pp. 370–378.
8. Drake, D.M., Feldman, W.C., and Jakosky, B.M., Martian Neutron Leakage Spectra, J. Geophys. Res., 1988, vol. 93, pp. 6353–6368.
9. Farmer, C.B. and Doms, P.E., Global and Seasonal Water Vapor on Mars and Implications for Permafrost, J. Geophys. Res., 1979, vol. 84, pp. 2881–2888.
10. Fedorova, A.A., Rodin, A.V., and Baklanova, I.V., MAWD Observations Revisited: Seasonal Behavior of Water Vapor in the Martian Atmosphere, Icarus, 2004a, vol. 171, no. 1, pp. 54–67.
11. Fedorova, A.A., Rodin, A.V., and Baklanova, I.V., Seasonal Cycle of Water Vapor in the Atmosphere of Mars as Revealed from the MAWD/ Viking 1 and 2 Experiment, Astron. Vestn., 2004b, vol. 38, no. 5, pp. 1–14 [Sol. Syst. Res. (Engl. Transl.), vol. 38, no. 5, pp. 421–433].
12. Feldman, W.C., Boynton, W.V., Tokar, R.L., et al., Global Distribution of Neutrons from Mars: Results from Mars Odyssey, Science, 2002, vol. 297, pp. 75–78.
13. Feldman, W.C., Prettyman, T.H., Boynton, W.V., et al., The Global Distribution of Near-Surface Hydrogen on Mars, Proc. Sixth Int. Conf. on Mars, 2003, Abstract #3218.
14. Forget, F., Hourdin, F., Fournier, R., et al., Improved General Circulation Models of the Martian Atmosphere from the Surface to Above 80 km, J. Geophys. Res., 1999, vol. 104, pp. 24155–21176.
15. Haberle, R.M., Pollack, J.B., Barnes, J.R., et al., Mars Atmospheric Dynamics as Simulated by the NASA AMES General Circulation Model. I – The Zonal-Mean Circulation, J. Geophys. Res., 1993, vol. 98, pp. 3093–3123.
16. Jakosky, B.M. and Farmer, C.B., The Seasonal and Global Behavior of Water Vapor in the Mars Atmosphere: Complete Global Results of the Viking Atmospheric Water Vapor Detector Experiment, J. Geophys. Res., Ser. B, 1982, vol. 87, no. 4, pp. 2999–3019.
17. Jakosky, B.M. and Barker, E.S., Comparison of Groundbased and Viking Orbiter Measurement of Martian Water Vapor: Variability of the Seasonal Cycle, Icarus, 1984, vol. 57, no. 3, pp. 322–334.
18. Jakosky, B.M. and Haberle, R.M., Year-To-Year Instability of the Mars South Polar Cap, J. Geophys. Res., 1990, vol. 95, pp. 1359–1365.
19. Jakosky, B.M., Mellon, M.T., Varnes, E.S., et al., Mars Low- Latitude Neutron Distribution: Possible Remnant Near- Surface Water Ice and a Mechanism for Its Recent Emplacement, Icarus, 2005, vol. 175, no. 1, pp. 58–67.
20. Jones, T.D., Arvidson, R.E., Guinness, E.A., et al., One Mars Year: Viking Lander Imaging Observations, Science, 1979, vol. 204, pp. 799–806.
21. Kuzmin, R.O., Ground Ice in the Martian Regolith, Water on Mars and Life, Tokano, T., Ed., Heidelberg: Springer, 2005, pp. 154–189.
22. Kuzmin, R.O. and Zabalueva, E.V., Polygonal Terrain on Mars: Preliminary Results of Global Mapping of Their Spatial Distribution, Lunar and Planet. Sci. Conf. XXXIV, 2003, Abstract #1912.
23. Kuzmin, R.O., Christensen, P.R., and Zolotov, M.Yu., Global Mapping of Martian Bound Water at 6.1 Microns Based on TES Data: Seasonal Hydration-Dehydration of Surface Minerals, Lunar and Planet. Sci. Conf. XXXV, 2004a, Abstract #1810.
24. Kuzmin, R.O., Zabalueva, E.V., Mitrofanov, I.G., et al., Regions of Potential Existence of Free Water (Ice) in the Near-Surface Martian Ground: Results from the Mars OdysseyHigh-Energy Neutron Detector (HEND), Astron. Vestn. , 2004b, vol. 38, no. 1, pp. 1–13 [ Sol. Syst. Res. (Engl. Transl.), vol. 38, no. 1, pp. 1–11].
25. Kuzmin, R.O., Zabalueva, E.V., Mitrofanov, I.G., et al., Seasonal Redistribution of Water in the Surfacial Martian Regolith: Results of the HEND Data Analysis, Lunar and Planet. Sci. Conf. XXXVI, 2005, Abstract #1634.
26. Kuzmin, R.O., Christensen, P.R., Zolotov, M.Yu., and Anwar, S., Mapping of Seasonal Bound Water Content Variations on the Martian Surface Based on the TES Data, Lunar and Planet. Sci. Conf. XXXVII, 2006, Abstract #1846.
27. Litvak, M.L., Mitrofanov, I.G., Kozyrev, A.S., et al., Seasonal Neutron-Flux Variations in the Polar Caps of Mars as Revealed by the Russian HEND Instrument Onboard the NASA 2001 Mars Odyssey Spacecraft, Astron. Vestn., 2003, vol. 37, no. 5, pp. 413–422 [ Sol. Syst. Res. (Engl. Transl.), vol. 37, no. 5, pp. 378–386].
28. Litvak, M.L., Mitrofanov, I.G., Kozyrev, A.S., et al., Comparison Between Polar Regions of Mars from HEND/ Odyssey Data, Icarus, 2006, vol. 180, no. 1, pp. 23–37.
29. Mellon, M.T. and Jakosky, B.M., The Distribution and Behavior of Martian Ground Ice During Past and Present Epochs, J. Geophys. Res., 1995, vol. 100, pp. 11781-11800.
30. Mellon, M.T., Feldman, W.C., and Prettyman, T.H., The Presence and Stability of Ice in the Southern Hemisphere of Mars, Icarus, 2004, vol. 169, no. 2, pp. 324–340.
31. Mitrofanov, I., Anfimov, D., Kozyrev, A., et al., Maps of Subsurface Hydrogen from High Energy Neutron Detector, Science, 2002, vol. 297, pp. 78–81.
32. Mitrofanov, I.G., Litvak, M.L., Kozyrev, A.S., et al., Search for Water in Martian Soil Using Global Neutron Mapping by the Russian HEND Instrument Onboard the US 2001 Mars Odyssey Spacecraft, Astron. Vestn., 2003, vol. 37, no. 5, pp. 400–412 [ Sol. Syst. Res. (Engl. Transl.), vol. 37, no. 5, pp. 366–277].
33. Mitrofanov, I.G., Litvak, M.L., Kozyrev, A.S., et al., Soil Water Content on Mars as Estimated from Neutron Measurements by the HEND Instrument Onboard the 2001 Mars Odyssey Spacecraft, Astron. Vestn., 2004, vol. 38, no. 4, pp. 291–303 [ Sol. Syst. Res. (Engl. Transl.), vol. 38, no. 4, pp. 253–257].
34. Montmessin, F., Forge, F., Pannou, P., et al., Origin and Role of Water Ice Clouds in the Martian Water Cycle as Inferred from a General Circulation Model, J. Geophys. Res., Ser. E, 2004, vol. 109, p. 10004.
35. Richardson, M.I. and Wilson, R.J., Investigation of the Nature and Stability of the Martian Seasonal Water Cycle with a General Circulation Model, J. Geophys. Res., 2002, vol. 107, p. 5031.
36. Richardson, M.I., Wilson, R.J., and Rodin, F.V., Water Ice Clouds in the Martian Atmosphere: General Circulation Model Experiments with Simple Cloud Scheme, J. Geophys. Res., 2002, p. 5064.
37. Rieder, R.R., Gellert, R., and Anderson, R.C., Bruckner J. Chemistry of Rocks and Soils at Meridiani Planum from the Alpha Particle X-Ray Spectrometer, Science, 2004, vol. 306, pp. 1746–1749.
38. Rodin, A.V. and Wlson, R.J., Seasonal Cycle of Martian Climate: Experimental Data and Numerical simulation, Kosm. Issled., 2006, vol. 44, no. 4, pp. 1–5 [Cosmic Res. (Engl. Transl.), vol. 44, no. 4, pp. 329–333].
39. Smith, M.D., The Annual Cycle of Water Vapor on Mars As Observed by the Thermal Emission Spectrometer, J. Geophys. Res., 2002, vol. 107, p. 19.
40. Smith, M.D., Interannual Variability in TES Atmospheric Observations of Mars During 1999–2003, Icarus, 2004, vol. 167, no. 1, pp. 148–165.
41. Squyres, S.W., Grotzinger, J.P., Aridson, R.E., et al., In Situ Evidence for An Ancient Aqueous Environment at Meridiani Planum, Mars, Science, 2004, vol. 306, pp. 1709–1714.
42. Titus, T.N., Kieffer, H.H., and Christensen, P.R., Exposed Water Ice Discovered Near the South Pole of Mars, Science, 2002, vol. 299, pp. 1048–1051.
43. Tokano, T., Water Cycle in the Atmosphere and Shallow Subsurface, Water on Mars and Life, Tokano, T., Ed., Heidelberg: Springer, 2005, pp. 191–216.
44. Tokano, T., Spatial Inhomogeneity of the Martian Subsurface Water Distribution: Implication from a Global Water Cycle Model, Icarus, 2003, vol. 164, no. 1, pp. 50–78.
45. Zent, A.P. and Quinn, R.C., Simultaneous Adsorption of CO2 and H2O Under Mars-Like Conditions and Application to the Evolution of the Martian Climate, J. Geophys. Res., 1995, vol. 100, pp. 5341–5349.
46. Zent, A.P., Howard, D.J., and Quinn, R.C., H2O Adsorption on Smectites: Application to the Diurnal Variation of H2O in the Martian Atmosphere, J. Geophys. Res., 2001, vol. 106, 14.667–14.674.
47. Zurek, R.W., Barnes, J.R., Haberle, R.M., et al., Dynamics of the atmosphere of Mars, in Mars, Kieffer, H.H.., Jakosky, B.M.., Snyder, C.W., et al., Eds., Tucson: Univ. Arizona Press, 1992, pp. 835–933.