The Mars Odyssey experiments in the third interplanetary network
K. Hurley a, I. Mitrofanov b, S. Charyshnikov b, V. Grinkov b, A. Kozyrev b, M. Litvak b, A. Sanin b, W. Boynton c, C. Fellows c, K. Harshman c, C. Shinohara c, R. Starr d
aSpace Sciences Laboratory, University of California, 7 Gauss Way, Berkeley, CA 94720-7450, USA
bInstitute for Space Research, Moscow, Russia
cLunar and Planetary Laboratory, University of Arizona Tempe, AZ, USA
dDepartment of Physics, The Catholic University of America, Washington, DC 20064, USA
Abstract - The Mars Odyssey spacecraft carries two experiments which are capable of detecting cosmic gamma-ray bursts and soft gamma repeaters. Since April 2001 they have detected approximately 200 bursts and, in conjunction with the other spacecraft of the interplanetary network, localized many of them rapidly and precisely enough to allow sensitive multi-wavelength counterpart searches. We present the Mars Odyssey mission and describe the two experiments.
DOI: 10.1016/j.asr.2004.12.062 - http://www.sciencedirect.com/science/article/pii/S0273117705000529
-------------------------------------------------------------------------------------------------------------------------------------------------
3. The gamma sensor head and the high energy neutron detector
-------------------------------------------------------------------------------------------------------------------------------------------------Interplanetary networks (IPNs) have played an important role in the studies of both cosmic gamma-ray bursts (GRBs) and soft gamma repeaters (SGRs) for over two decades. Indeed, until the launch of BeppoSAX in 1996, the only way to derive arcminute positions for these objects was by comparing their arrival times at distant spacecraft. The current (third) IPN was formed when the Ulysses spacecraft was launched in 1990. Over 20 spacecraft have participated in the IPN since then, and the latest interplanetary mission to join the network is Mars Odyssey. Today, the IPN comprises the Ulysses, Konus-Wind, Ramaty High Energy Solar Spectroscopic Imager, High Energy Transient Explorer, and Mars Odyssey (MO) missions and experiments, and, with a detection rate of about 200 events/year, is responsible for most GRB and SGR detections and localizations. As a distant point in the network, MO plays a crucial role: without it, only localizations to annuli or large error boxes would be possible. The triangulation, or arrival-time analysis method for localizing bursts has been presented elsewhere ( [Hurley et al., 1999a] and [Hurley et al., 1999b] ). Here, we concentrate on the properties of the two MO experiments which make burst detection possible. (An expanded version of this paper is in preparation.) We note that this is the fifth attempt, and the first successful one, to place a GRB detector in Mars orbit; the four previous attempts, aboard the Phobos 1 and 2 (Sagdeev and Zakharov, 1990), Mars Observer (Metzger et al., 1992), and Mars '96 (Ziock et al., 1997) missions, met with limited or no success due to mission failures.
The Mars Odyssey mission is an orbiter whose objective is to provide a better understanding of the climate and geologic history of Mars. It was launched on 2001 April 7, and after a 6 month cruise phase, reached Mars on 2001 October 24. The mission then entered an aerobraking phase to circularize the orbit which lasted until 2002 January. At the end of this phase, the spacecraft was orbiting the planet every 1.964 h at an altitude between 370 and 432 km. The prime scientific mission then commenced, and continued through 2004 August; the mission is now in its extended phase.
The spacecraft is shown in Fig. 1. In its present orbit, Mars subtends approximately 30% of the sky at the Odyssey spacecraft. In general, the instruments are powered on continuously, and almost 100% of the data is downlinked through the Deep Space Network during daily tracking passes. A more complete description of the mission has appeared in Saunders et al. (2004).
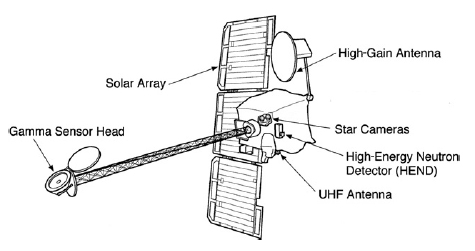
Fig. 1. The Mars Odyssey spacecraft, showing the positions of the HEND and GRS experiments, on the body of the spacecraft and on the boom, respectively.
3. The gamma sensor head and the high energy neutron detector
The Gamma-Ray Spectrometer (GRS) is an instrument suite which includes two detectors with GRB detection capabilities, the gamma sensor head (GSH), and the High Energy Neutron Detector (HEND). The principal objective of the GRS experiment is the determination of the elemental abundances on Mars. The GSH consists of a 6.7 cm diameter × 6.7 cm high (cross-sectional area to GRBs ∼40 cm2) right circular cylindrical germanium detector which is passively cooled and mounted on a boom extending 6 m from the body of the spacecraft (see Fig. 1). It records energy spectra between ∼50 keV and 10 MeV in a low time resolution mode (∼20 s) until triggered by a burst. It then records GRB time histories in a single energy channel with 32 ms resolution for 19.75 s, and can retrigger immediately thereafter. The boom extension and detector cooling did not take place until after the end of the aerobraking phase, and thus the experiment did not collect useful GRB data until then. The in-orbit background rate is ∼100 counts/s in the GRB energy channel, but it undergoes variations due to numerous causes. In order of decreasing importance, these are: (a) the albedo from the Martian surface due to the cosmic gamma-ray background, which is different for different regions of the planet; (b) seasonal changes on ∼month timescales, such as CO2 condensation; (c) solar proton events. The GSH is shown in Fig. 2. More details may be found in Boynton et al. (2004).
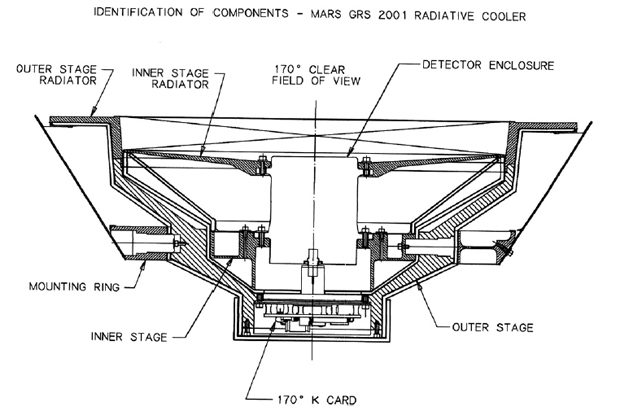
Fig. 2. Cross-section of the gamma sensor head, showing the germanium detector and the cooler. The upper part of the head faces into space, and Mars is toward the bottom.
The burst detection portion of the HEND experiment is based on two optically separate scintillation detectors (Fig. 3). The first is a cylindrical stilbene crystal with a diameter of 30 mm and a height of 10 mm, which is used for the detection of high energy neutrons, and records gamma-rays as a by-product. These counts are measured continuously in the 350–3000 keV range with a time resolution of 1 s. The second detector is a cylindrical CsI(Tl) anti-coincidence well surrounding the stilbene, whose thickness is 10 mm, whose outer diameter is 50 mm, and whose height is 49 mm. Thus, its cross-sectional area to GRBs varies between 19.6 and 24.5 cm2. In triggered mode, counts from the CsI are recorded in the ∼30–1300 keV energy range with a time resolution of 250 ms, and these data are used for triangulation. The upper and lower energy limits are only approximate, since the light collection in the cylindrical crystal depends upon the photon interaction point. HEND is mounted on the body of the spacecraft. The in-orbit background rates are ∼5 counts/s for the inner stilbene detector, and ∼130 counts/s for the CsI anticoincidence. Both these rates undergo variations for the same reasons as the GSH, and in addition, because of HEND's lower energy threshold, due to trapped particles. For example, for a period of approximately 7 months starting on November 11, 2002, the background variations along the orbit increased from their nominal value of about a factor of 2 to a factor of 30. The cause of this increase is suspected to be trapped particles. During this time, the duty cycle for GRB detection decreased by a factor of 3.4. These large variations disappeared around June 9, 2003. Occasionally they reappear rather reduced in strength. More details of the instrument may be found in Boynton et al. (2004).
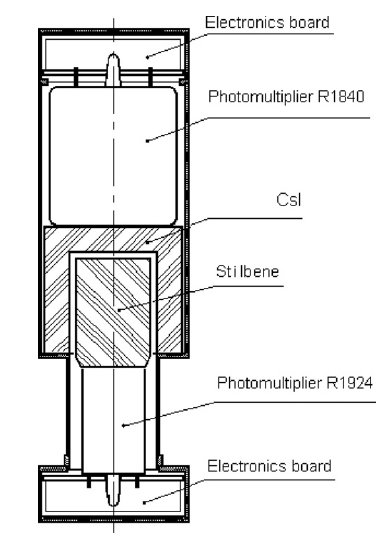
Fig. 3. The High Energy Neutron Detector (HEND) experiment. The detector axis, indicated by the center line, points towards the surface of Mars. The field of view of the CsI anticoincidence is mainly limited by the Mars horizon; the body of the spacecraft blocks a small fraction of the sky with relatively low atomic number materials.
HEND was turned on during the cruise phase and detected its first confirmed GRB on 2001 May 8. In the 1161 days which followed, HEND and/or GRS have detected >200 GRBs or SGRs which have been confirmed by detection aboard at least one other IPN spacecraft. Thus the average burst detection rate during the cruise and orbital phases is about one burst every 5.8 d. This number is an average over the entire period, and does not take high background periods, or times when the experiments were turned off, into account. The true rate would therefore be higher. Initially, the spacecraft timing was not determined accurately during the cruise phase and no triangulation results were announced. (These data have since been reprocessed for accurate timing.) Thus, the Odyssey mission became most useful to the IPN when it entered its orbital phase; at that point its separation from Earth was large enough for the triangulation method to produce small error boxes, and the timing was known to good accuracy in real time. In this phase, counting only those events which were observed by three widely separated spacecraft and localized to small error boxes, and which were localized rapidly enough for sensitive multi-wavelength counterpart searches, the rate has been about 2.7/month.
The GRS and HEND experiments aboard the Mars Odyssey spacecraft have been successfully integrated into the third interplanetary network. In this configuration, the IPN is producing rapid, precise GRB localizations at the expected rate, and should continue to do so for the forseable future. With the advent of newer missions, with much greater sensitivities, and which are capable of independently determining GRB positions to better accuracies and with shorter delays, the question inevitably arises whether it is useful to maintain an IPN. There are several arguments in favor of this. The new missions achieve their accuracy and sensitivity at the expense of sky converage; typically they view only 10–20% of the sky, while the IPN is isotropic. Thus, first, IPNs serve as continuous monitors of SGR activity throughout the galaxy, which the newer missions do not. Second, IPNs will detect strong bursts at a rate which is 5–10 times greater than that of the newer missions. Therefore they should continue to serve a useful role in GRB and SGR studies for years to come.
K.H. is grateful for support under NASA's LTSA and Odyssey Participating scientist programs. We also acknowledge the design work by John Laros which made GRB detection possible.
Boynton et al., 2004 W. Boynton, W. Feldman and I. Mitrofanov, et al. The Mars Odyssey gamma-ray spectrometer instrument suite. Space Sci. Rev., 110 (2004), pp. 37–83.
Hurley et al., 1999a K. Hurley, M. Briggs and R.M. Kippen, et al. The Ulysses supplement to the BATSE 3B catalog of cosmic gamma-ray bursts. Ap. J. Supp., 120 (1999), pp. 399–408.
Hurley et al., 1999b K. Hurley, M. Briggs and R.M. Kippen, et al. The Ulysses supplement to the BATSE 4Br catalog of cosmic gamma-ray bursts. Ap. J. Supp., 122 (1999), pp. 497–501.
Metzger et al., 1992 A. Metzger, W. Boynton, J. Laros and J. Trombka, Burst detection capability of the Mars Observer gamma-ray spectrometer, AIP Conf. Proc., vol. 265 ,in: W. Paciesas, G. Fishman, Editors , Gamma-Ray Bursts, AIP Press, New York (1992), pp. 353–358.
Sagdeev and Zakharov, 1990 R. Sagdeev and A. Zakharov, A brief history of the expedition to Phobos. Sov. Astron. Lett., 16 2 (1990), pp. 125–128.
Saunders et al., 2004 R. Saunders, R. Arvidson and G. Badhwar, et al. 2001 Mars Odyssey mission summary. Space Sci. Rev., 110 (2004), pp. 1–36.
Ziock et al., 1997 K. Ziock, J. Bixler and T. Cline, et al. The gamma-ray burst monitor for the Russian Mars 1996 Mission. IEEE Trans. Nuc. Sci., 44 4 (1997), pp. 1692–1701.